This is an appendix to: Huser et al. 2023. Handbook – a decision-support tool for measures against internal phosphorus loading in lakes.
Download pdf-version of appendix 6
Introduction
Phosphorus is an essential substance for all photosynthesising organisms. In lakes, usually, phytoplankton form the basis of the food web. Phytoplankton production increases when the phosphorus influx to lakes is elevated by means of municipal and industrial wastewater or nutrient diffusion from agricultural land, for example, a phenomenon known as eutrophication. Eutrophication of lakes began to be recognised as a growing environmental problem in the late 1960s, and various societal measures were implemented to reduce the input of phosphorus by introducing phosphorus precipitation at municipal wastewater treatment plants, for example. These measures have been effective in many cases, but not all. What is known as internal loading is one reason some lakes continue to exhibit high nutrient levels, despite the reduction of the external phosphorus influx to the lake via inflowing watercourses and direct point source discharges. A significant amount of phosphorus is normally fixed in the bottom sediment of lakes in biologically unavailable forms, but under certain conditions this process becomes less efficient and bioavailable phosphorus continues to circulate between water and sediment year after year, thus stimulating algal production. Societal measures against this internal pressure have been discussed increasingly in recent years, and specific measures have been implemented in some places.
Life cycle assessment (LCA) allows the effectiveness of environmental protection measures to be evaluated based on their overall environmental impact, considering not only their positive impact on the environment but also their potentially adverse environmental impact. An LCA, sometimes referred to as a cradle-to-grave analysis, evaluates a technical system or process in terms of all its components, such as the natural resources consumed in manufacturing, transport, energy consumption during use and resource consumption during final disposal. Environmental impacts are quantified for different impact categories, such as acidification potential, climate impact and eutrophication potential. IVL Swedish Environmental Research Institute has a long tradition of utilising the LCA tool in holistic assessments of various environmental protection measures. LCA calculations are presented below, specifically in respect of the climate impact of various measures aimed at reducing internal loading of phosphorus in lakes.
Methodology
Life cycle assessment (LCA) is a summary and evaluation of relevant influxes and outfluxes from a product system or process and an evaluation of the potential environmental impacts of the product system throughout its life cycle (ISO 14040:2006 and 14044:2006). Influxes and outfluxes refer to the use of natural resources and the generation of emissions and residual products associated with the system, respectively. The life cycle constitutes a technical system consisting of processes and transport at all stages from the extraction of natural resources to the final disposal of the product and the elimination of residual products (waste disposal and recycling). LCA is sometimes referred to as “cradle-to-grave analysis” (Fig. 1).
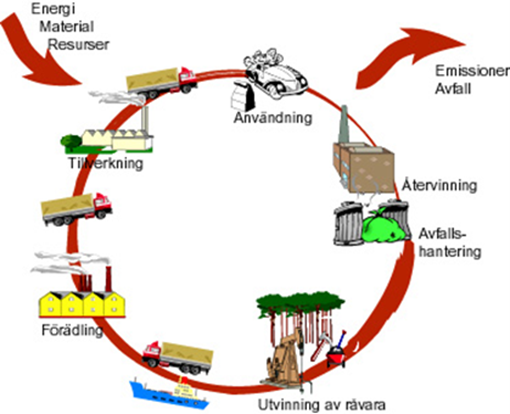
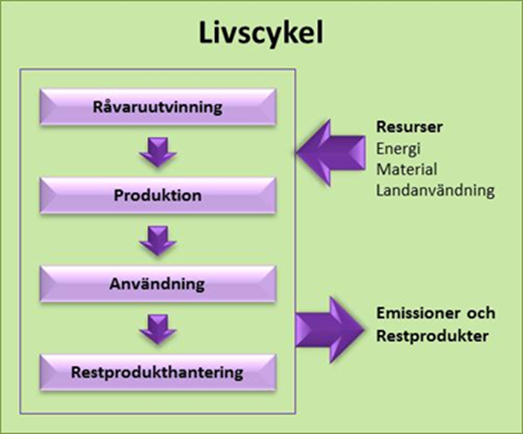
In theory, there are a number of measures that can be used to reduce internal loading, ranging from physically excavating sediments or diverting phosphorus-rich bottom water to depleting white fish stocks or using precipitation chemicals in an analogous way to wastewater treatment plants to precipitate phosphorus in non-bioavailable forms. The most proven method, both internationally and in Sweden, is to precipitate the phosphorus in the bottom sediments by adding an aluminium salt to the water body or bottom sediments of the water area in a form where it will not diffuse back into the water body but be buried in a stable compound. This is a process that occurs naturally in most aquatic environments. Aluminium is the fourth most abundant element in the Earth’s crust, but in areas with an excess of phosphorus, these binding particles are simply not present in sufficient quantities to combine with all the phosphorus available. About ten lakes in Sweden have been treated in this way with generally satisfactory results, and in North America about a hundred lakes have been treated with aluminium since the 1960s.
Another method is to heavily reduce fish stocks, especially species such as bream, roach, smelt and ruffe by means of depletion. A certain amount of phosphorus is forcibly removed from the lake by means of heavy fishing pressure. Strictly speaking, reduction fishing is not a measure against internal loading but a measure to reduce the amount of phosphorus in a water system regardless of whether the load comes from external or internal sources. However, we include it here as it can be used to reduce the phosphorus content of internally loaded lakes. Dredging of bottom sediments is a relatively common measure in an environmental management context when it comes to cleaning up delimited areas to remove environmentally hazardous substances. One technique that has been discussed and started to be tested in recent years is what is known as low flow dredging, which involves the gradual suction dredging of surface sediments over a slow period and dispersing the resulting dredged material onto surrounding arable land as a soil improver. Overall, there are a number of potential methods to reduce internal loading of phosphorus that differ considerably in terms of technical design, including the resources they require, and the resulting carbon dioxide emissions generated (Fig. 2). The following are evaluated below:
- Aluminium treatment of sediments
- Conventional dredging
- Low flow dredging
- Reduction fishing
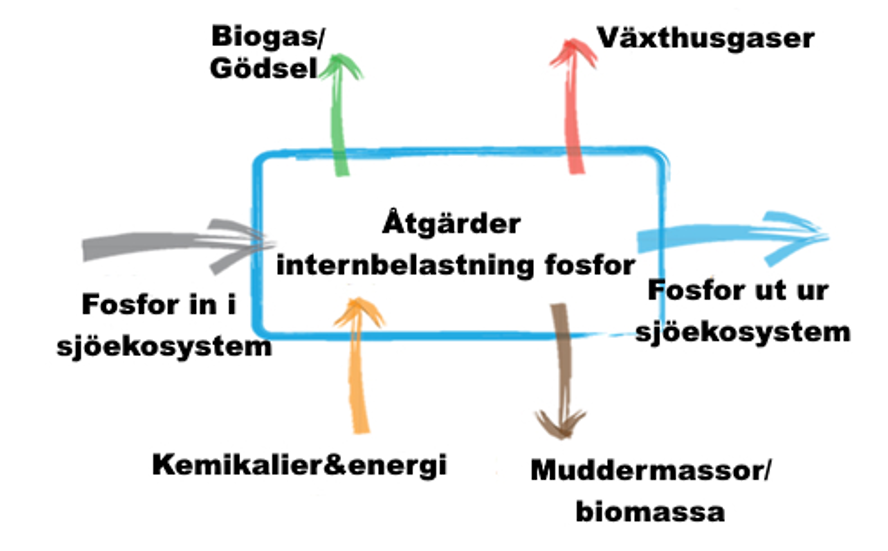
The functional unit for the calculations has been 1 kg of potentially bioavailable phosphorus (Mob-P), i.e., all calculations have been normalised to answer the question of how much carbon dioxide each measure generates to withdraw 1 kg of Mob-P from the water body in question. When calculating transport distances, we have assumed that the measures are implemented in an urban lake in central Sweden. Previous work at IVL Swedish Environmental Research Institute (Karlsson et al., 2012; Karlsson et al., 2019) has provided a starting point for the calculations.
Results
Aluminium treatment
PAX XL 100 (polyaluminium chloride) can be applied to lake water bodies or bottom sediments from a boat to remove phosphorus. It is assumed below that 100 kg of PAX XL 100 is required for every kg of fixed phosphorus (Karlsson et al., 2019). The precipitation chemical is produced in Helsingborg and transported from Helsingborg to central Sweden (700 km) by lorry. It is dispersed at sea from a petrol-powered boat that uses 0.55 liters of petrol to spread 100 kg of PAX XL 100. The contribution to climate impact from precipitation of phosphorus using PAX XL 100 under these assumptions is then as stated in Table 1.
kg CO2e/kg of P | |
Production, including transport | 50 |
Transport | 7 |
Dispersal | 3 |
Total | 60 |
By way of comparison, municipal wastewater treatment plants treat wastewater to remove phosphorus in an analogous way to the treatment of lakes with precipitation chemicals. One way to reduce the phosphorus load on recipients is to increase phosphorus precipitation at wastewater treatment plants. Thus, resources in the form of energy and precipitation chemicals are required to reduce phosphorus emissions. Based on experience and previous projects (Åmand et al., 2016), increased phosphorus removal at a municipal wastewater treatment plant with normal technical standards is typically estimated to lead to an increased climate impact of about 40 kg CO2e/kg of phosphorus removed.
Conventional dredging
Conventional dredging, such as mechanical and hydraulic dredging, is often used for excavation of lake beds, and sometimes for the removal of contaminated sediments as well (Elander & Jersak, 2017). The choice of dredging method is often linked to the type of lake bed: for example, mechanical dredging (also known as dredging bucket or trench dredging) is suitable for solid type lake beds with rocks and boulders, while hydraulic dredging (also known as suction dredging) is more suitable for loose lake beds such as mud, loose clay, sand, or gravel. Depth, dredged material volume, contaminant content and cost are other criteria to be considered when choosing a dredging method (Swedish Agency for Marine and Water Management, 2018).
In the case of suction dredging, sediment mixed with water is sucked into the dredger in what is known as a slurry. This slurry is then transported via pipelines to a designated site where it can be dumped or dewatered. Polymers are sometimes used to accelerate the dewatering of dredged material, and polyaluminium chloride (PAC) can be used to precipitate the phosphorus in the slurry. Figure 3 shows the processes that were used to perform the LCA for conventional dredging.
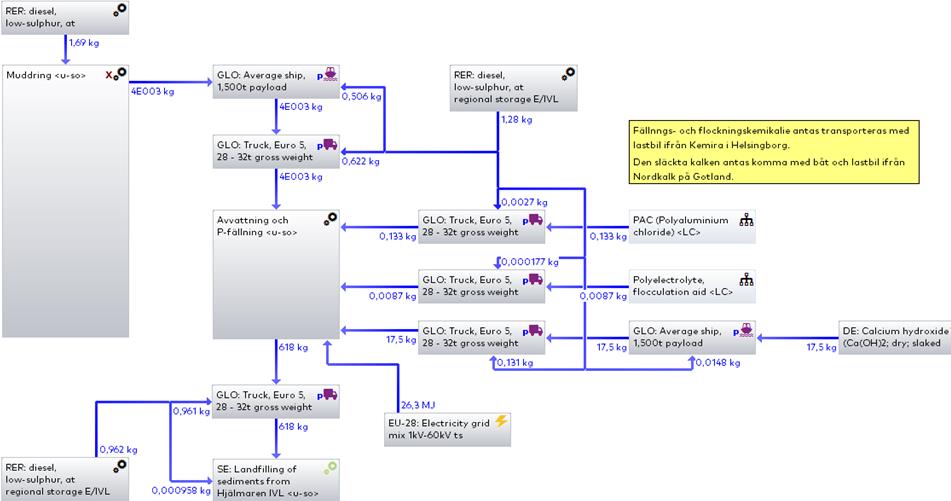
The results of the LCA for an average conventional dredging operation are shown in Table 2. The calculation used pump flows and energy consumption from a dredging operation in the Oskarshamn harbour basin (Oskarshamn, 2016). It was also assumed that the dredger was transported 15 kilometers by barge, and that chemicals were used to dewater the dredged material and precipitate the phosphorus. The dredged material was also assumed to have been transported 30 km by lorry to a landfill site.
kg CO2e/kg of P | |
Chemicals (dewatering, precipitation, flocculation) | 16 |
Transport during dredging | 4 |
Transport of dredged material to a landfill site | 2 |
Other | 3 |
Total | 25 |
Low flow dredging
Low flow dredging is a variant of suction dredging where a dredging unit floats above the bottom and sucks up the bottom material (Swedish Agency for Marine and Water Management, 2018). This method is relatively new and still under development. In the case of conventional dredging, the aim is usually to excavate the bottom sediments quickly and so the mass flow is high. In the case of low flow dredging, the mass flow is lower, and dredging can be controlled more carefully. Another advantage of the method is that it produces significantly less particle dispersion compared to conventional dredging methods and is therefore kinder to the environment (Swedish Agency for Marine and Water Management, 2018).
The lower mass flows mean that the energy consumption is less per unit of time, which opens the opportunity to use renewable fuels. A low flow dredging operation conducted in Lake Ralången in the county of Jönköping (Lindell et al., 2022) used an electrically powered dredger and a pump that was powered by on-site solar panels for much of the operation.
In the case of low flow dredging, only the top layer of the sediment is removed as this is where potentially bioavailable forms of phosphorus are most abundant. Another positive side effect of removing this top layer of sediment is the removal of oxygen-consuming material, which can improve the structure of the soil on arable or forest land. The mass flow rate is lower than with conventional dredging, and so it may be easier to control the depth of dredging in the sediments.
To compare the climate impact of low flow dredging with other phosphorus reduction methods in lakes, we have performed an LCA based on the dredging of Lake Ralången (Lindell et al., 2022). This LCA includes transport to and from the lake and dewatering of the sediments.
Dredging took place as follows. Firstly, a petrol-powered barge deployed the dredger a short distance from the shore. The dredger was then winched in towards the shore before being towed out again by the barge. This procedure was repeated until the predetermined bottom surface was dredged. The winch was powered by a diesel-powered generator on land.
The dredged material was transported from the barge via a hose to an on-site sedimentation basin with a drilled filter well. The flow from the dredger was approximately 7 m3/hour, and a total of approximately 750 m3 of slurry (sediment and lake water) was pumped. The sedimentation basin was constructed from concrete bricks and dug into the ground using an excavator (the excavator was used for a couple of hours in total). The dredged material was cleaned via geomembranes and sand filters, and the filtrate was pumped back into the lake when it was as clean as or cleaner than the lake water. Some 50% of the weight of the dredged material was returned to the lake, and 50% remained in the sedimentation basin.
During dredging, the lake water was pumped back into the lake using a pump powered by the generator. When dredging was completed and no new dredged material was added, a solar-powered pump was connected to the filter well which continued to pump back the lake water: no energy was now added other than that from the solar panels. No chemicals were used for dewatering. All sediment from the dredging operation ended up in six containers, equaling about 29 kg of phosphorus. The process flow of the LCA for low flow dredging is shown in Figure 4. The carbon footprint is shown in Table 3.
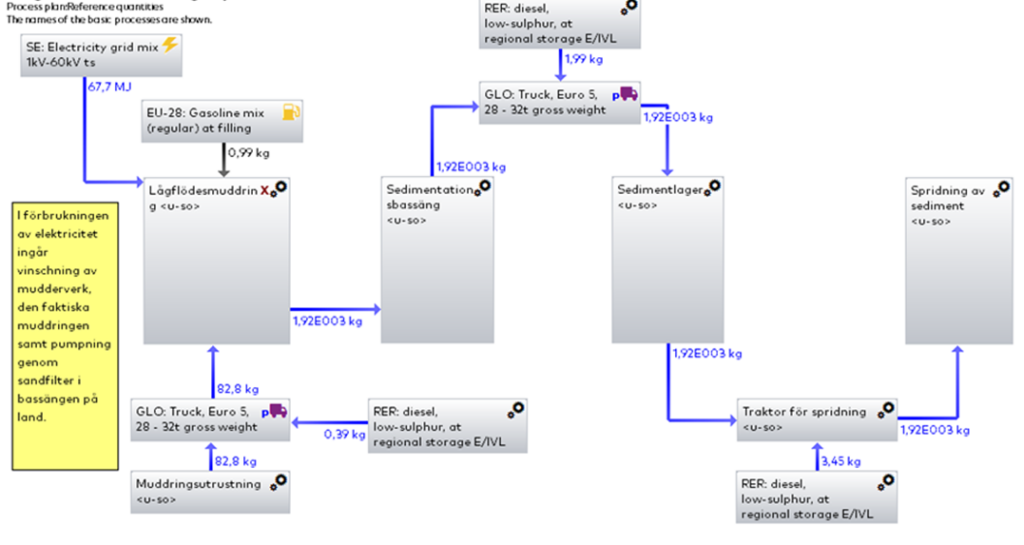
kg CO2e/kg of P | |
Transport of dredged material for dispersal | 7 |
Dispersal of dredged material | 2 |
Dredging | 1 |
Other | 2 |
Total | 12 |
Reduction fishing
The aim of reduction fishing, which involves extraction of large numbers of various white fish species, is to harvest nutrient salts, mainly phosphorus, by removing fish that have lived and grown up in the water body, and to change the species composition to favour large predatory fish. A higher proportion of large predatory fish results in stronger predation pressure on small plankton and benthivorous fish. If the density of these species is reduced, there may be more zooplankton and more benthic animals, with subsequent grazing on phytoplankton and benthic algae. This results in greater water transparency and reduced fouling on rocks and macroalgae such as bladderwrack, which in itself is a reduction of the symptoms of eutrophication. However, only the direct phosphorus reduction effect is considered below.
If a decision to made to digest the catch obtained after reduction fishing, CO2 emissions are generated during boat transport to and from the fishing grounds, when freezing the catch and during transport of the fish to a biogas plant. The biogas plant generates a fuel, methane gas, which in this analysis is regarded as a negative CO2 emission as it can replace fossil fuels such as petrol as a vehicle fuel. In a trial at KTH Royal Institute of Technology (Shi, 2012) involving the digestion of sewage sludge, fish offal and by-catches from commercial fishing, a yield corresponding to 0.5 m3 if gas per kilogram of wet weight of fish was obtained. With this as a starting point and an assumption that the catch needs to be transported 50 km by lorry to a biogas plant, a negative carbon footprint is achieved as shown in Table 4. If the catch from reduction fishing were to be marketed as food or animal feed instead, there is also a potential carbon saving in that other products will be replaced.
kg CO2e/kg of P | |
Biogas production | -25 |
Transport | 1 |
Total | -24 |
Concluding discussion
According to Table 5 and Figure 4, the highest carbon footprint per kilogram of phosphorus removed is generated by aluminium treatment, followed by conventional dredging and low flow dredging, while reduction fishing generates a carbon saving by utilising the catch as biogas. Compared with measures to improve treatment at municipal treatment plants with normal technical standards (Åmand et al., 2016), it can be concluded that measures against internal loading are within the same order of magnitude in terms of carbon footprint.
Measure | kg CO2e/kg of P |
Aluminium treatment | 60 |
Conventional dredging | 25 |
Low flow dredging | 12 |
Reduction fishing | -24 |
Measures at municipal wastewater treatment plant | 40 |
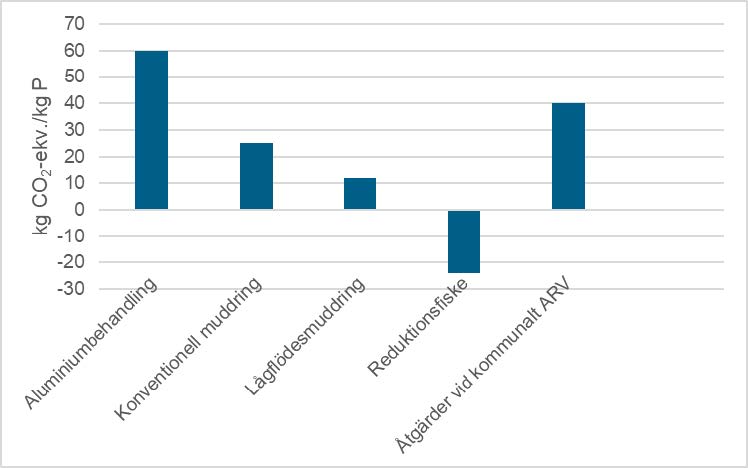
As a further comparison, the annual carbon dioxide emissions from consumption by the Swedish population are estimated at 8 tonnes/person and year (https://www.naturvardsverket.se/data-och-statistik/konsumtion/vaxthusgaser-konsumtionsbaserade-utslapp-per-person). Aluminium treatment of a lake surface 100 ha in area generates a climate impact of 50 tonnes of CO2e, or annual emissions for just over six people.
Another aspect of climate impact, which was beyond the scope of this study, is how the measures themselves affect the carbon balance in the lakes. In lakes with stressed oxygen conditions, measures against internal loading can improve oxygen conditions along the bottom, which could lead to a higher proportion of the organic matter deposited along the bottom being broken down and released as carbon dioxide and a lower proportion being permanently fixed in the sediments. That said, improved oxygen conditions could lead to reduced methane formation, which in the short term is a more potent greenhouse gas.
References
Elander, P. & Jersak, J., 2017. (In Swedish) Fiberbankar i Norrland – Metoder för efterbehandling av fibersediment. County Administrative Boards report 2017:1.
Swedish Agency for Marine and Water Management, 2018. Swedish Agency for Marine and Water Management report 2018:19: (In Swedish) Muddring och hantering av muddermassor Vägledning och kunskapsunderlag av 11 och 15 kap. miljöbalken.
Karlsson, M., Malmaeus, M., Baresel, C., Sivard, Å., Ericsson, T. & Grahn, O., 2012. (In Swedish) Kostnadseffektivitet i åtgärder mot övergödning – Fallstudie Gävle fjärdar, IVL-rapport B2078.
Karlsson, M. & Malmaeus, M. & Rydin, E., 2019. (In Swedish) Åtgärder mot internbelastning av fosfor i Hjälmaren kostnad, nytta och konsekvenser. IVL report C381.
Lindell, M., Carlsson, F., & Ekström, M., 2022. (In Swedish) Återföring av näringsrika ytsediment till produktionsmark – Erfarenheter av muddring, pelletsproduktion samt testodling. Jönköping County Administrative Board, Bulletin 2022:09.
Municipality of Oskarshamn, 2016. (In Swedish) Saneringen av Oskarshamns hamnbassäng., 2016 Projekt och erfarenhetsrapport 1996–2015, 2016:1.
Shi, C., 2012. Potential biogas production from fish waste and sludge. M.Sc. thesis. Department of Land and Water Resources Engineering. Royal Institute of Technology (KTH). TRITA LWR Degree project 12:37.
Åmand, L., Andersson, S., Oliveira, F., Rahmberg, M., Junestedt, C., Arnell, M., 2016. (In Swedish) Nya utsläppskrav för svenska reningsverk – effekter på reningsverkens totala miljöpåverkan. IVL report B2246.