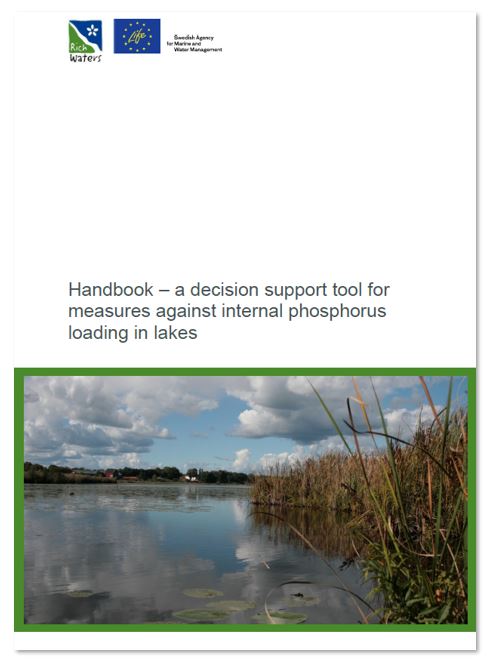
Introduction
To achieve good ecological status regarding eutrophication in Swedish lakes and coastal waters, it is in many cases insufficient to reduce only the phosphorus load from external sources such as wastewater treatment plants, individual sewage systems, agriculture and industry. In such cases elevated internal loading of phosphorus from lake sediments may constitute a significant proportion of the total phosphorus load and will also need to be addressed.
Measures against internal loading are, however, no substitute for measures against external nutrient loads, as the external nutrient load has to be reduced to a sufficiently low level for measures against internal loading measures to remain effective in the long term. Even if a successful measure against internal loading results in a temporary reduction of phosphorus concentrations, the external load will determine the eutrophication situation in the water body in the long term. It may therefore be necessary to implement measures against both external inputs and internal loading to the same lake.
The extent to which elevated internal loading occurs is not known in detail, but it is likely to be a problem in many of the hundreds of lakes and coastal waters in Sweden affected by eutrophication. Investigating in detail the waters in which internal loading measures would be effective, and if so, what those measures would be, would be an almost insurmountable task. Against this background, this handbook presents a decision support tool, in four steps, developed by Brian Huser, Mikael Malmaeus, Magnus Karlsson, Robert Almstrand and Ernst Witter with assistance from many partners in the LIFE IP project Rich Waters, as well as several county administrative boards participating in an associated project funded by the Swedish Agency for Marine and Water Management (SwAM).
The decision support tool is designed to be used by water authorities, county administrative boards, municipalities, water organisations and other stakeholders in order to determine when and how to address internal loading. In the first step, a simple assessment of risk for elevated internal loading can, in most cases, be made on the basis of data already collected in the assessment of the ecological status of lakes and coastal waters. Users can then proceed in three further steps that often require additional data to reach a decision if measures against internal loading are warranted, and which measures may be suitable. The hope is that the amount of work with respect internal loading will become more manageable, and that as much emphasis as possible will be placed on waters where action is needed most.
Although this manual is complete and the information in it has been reviewed by the authors, the development of the decision support tools is an ongoing process. New, updated versions of the guide are therefore expected to be produced. The current version is largely – but not exclusively – based on data from lakes in southern and central Sweden. We hope to further develop the tools for coastal waters as well as lakes in northern Sweden where we currently lack sufficient data.
We welcome your comments on the handbook and its appendices. These can be sent to the authors:
Brian Huser, Swedish University of Agricultural Sciences – brian.huser@slu.se (Part I. Decision support tool steps 1 and 2, and Appendices 1–5 and Part II – Detailed description of actions)
Mikael Malmaeus, IVL – mikael.malmaeus@ivl.se (Part I. Decision support tool Step 3)
Magnus Karlsson, IVL – magnus.karlsson@ivl.se (Part I. Decision support tool Step 4, Part II. Detailed description of measures)
Robert Almstrand, HaV – robert.almstrand@hav.se (Part I. Decision support tool Step 4, information on funding opportunities, and Part II. Detailed description of measures)
Ernst Witter, Örebro County Administrative Board – ernst.witter@lansstyrelsen.se (general questions, and Appendices 1–5)
Purpose
The purpose of this handbook is to:
- describe the problem of internal phosphorus loading
- provide guidance on when it may be appropriate to implement measures against internal loading
- provide information on the measures currently in use
- provide information on how actions can be funded.
Structure
Part I focuses on internal phosphorus loading and begins by describing this problem. This is followed by a decision support tool aimed at supporting risk assessment, sampling, quantification and modelling of phosphorus dynamics in inland lakes. Detailed methodology can be found in Appendices 1–5.
The decision support tool is followed in Part I by an overview of measures in the aquatic environment that counteract the release of phosphorus from sediment and measures that directly remove sediment and nutrient-rich bottom water. Part II of the handbook, “Detailed description of measures”, develops the descriptions of the various measures, their advantages and disadvantages, appropriate location and a selection of documented experiences.
The handbook consists of a main document in two parts and six supporting appendices:
- Part I. Identification of lakes with elevated internal loading and selection of measures
- Part II. Detailed description of measures
- 6 appendices:
Part I. Identification of lakes with elevated internal loading and selection of measures
Authors:
Brian Huser, Swedish University of Agricultural Sciences, Uppsala
Mikael Malmaeus, IVL, Stockholm
Magnus Karlsson, IVL, Stockholm
Robert Almstrand, Swedish Agency for Marine and Water Management, Gothenburg
Ernst Witter, Örebro County Administrative Board
1 What is internal loading?
Eutrophication of lakes and the coastal waters of the Baltic Sea is largely determined by the availability of phosphorus. The phosphorus dynamics in a lake or coastal areas are an interaction between external and internal fluxes. External phosphorus inputs from the catchment area occur naturally via waterways, but human activities such as agriculture and effluent from sewage and industry can increase the inputs and sometimes cause eutrophication in lakes and seas. Sedimentation, mixing and diffusion of phosphorus from sediments are important internal fluxes. Figure 1 illustrates the phosphorus dynamics in a lake. The dynamics are similar in coastal waters, but here the exchange of water and phosphorus between the coastal bay and the sea are also important fluxes.
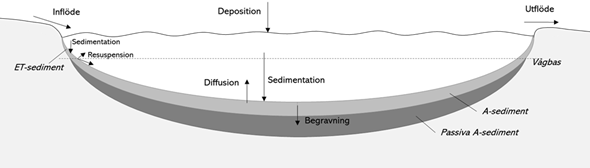
Internal loading means that phosphorus from the bottom sediments is transported to the water phase by diffusion or resuspension (see Figure 1). Both physical factors such as wind, and chemical factors such as redox potential, influence the extent to which phosphorus is released from sediments to water. The phosphorus present in the sediments originates from sedimentary material and has thus been initially introduced to the system from outside the water body via external inputs. Internal loading is a natural element of phosphorus dynamics, but if large amounts of phosphorus have accumulated in the sediments due to high external inputs, internal loading can become elevated, i.e. greater than in lakes unaffected by past external phosphorus inputs from human activity. In eutrophic lakes, it is common for elevated internal loading to occur long after external inputs have been reduced, as it can take a long time, sometimes decades or longer, for the excess phosphorus accumulated in the bottom sediments to become depleted. Some forms of phosphorus are particularly prone to diffusion and are collectively referred to as mobile phosphorus. Mobile phosphorus includes iron-bound phosphorus and some organic forms of phosphorus (see Appendix 3). Non-mobile phosphorus bound to particles can re-enter the water by means of resuspension as well, but only part of this phosphorus is biologically available.
By definition, there is a difference between gross internal load and net internal load. Gross internal load is the magnitude of the phosphorus flux from sediments, while net internal load is the gross internal load minus the sedimentation of phosphorus on the bottom. In other words, a positive net internal load means that the amount of phosphorus accumulating in the water body or leaving the lake is greater than the amount entering the lake. Internal loading varies throughout the year and is often highest in summer. In un- or moderately affected systems, there is usually no net internal load on an annual basis as more phosphorus is deposited than is released from the bottom. This is referred to as phosphorus retention or fixation.
The gross internal load may be elevated even if there is no net internal load. This then leads to the amount of phosphorus in the water being higher than it would be in an unaffected system. However, it is not easy to determine what constitutes a natural internal load. In purely conceptual terms, elevated internal loading can be considered to describe the difference between the internal loading of an affected system and a system unaffected by anthropogenic phosphorus inputs.
1.1 Internal phosphorus loading in Swedish lakes
There are about 600 lakes in Sweden that do not achieve at least good nutrient status.[1] The extent to which elevated internal loading contributes to eutrophication of these lakes is generally not known. Although internal loading is likely to be elevated in many eutrophied lakes, this does not automatically mean that measures are required to reduce it. In other cases where measures already have reduced external phosphorus inputs, however, reducing the internal load may be crucial for reducing eutrophication. To assess the contribution of internal loading to eutrophication of a lake or coastal water, a site-specific assessment of the phosphorus dynamics needs to be carried out. In a number of Swedish lakes and coastal areas, problems with eutrophication have prompted more detailed studies and measures have been carried out to address this problem. In most cases measures against external loading were implemented initially, but in some cases these were not enough to reduce phosphorus concentrations in the water sufficiently. This has sometimes prompted further action including implementation of measures to reduce the internal load. This has often resulted in improved water quality, but the duration of the measures has varied greatly. Insufficient knowledge of the external and internal phosphorus fluxes (see Figure 1), and how these are affected by the measures, is often the reason why the anticipated effect of measures fails to materialise or does not turn out to be sustainable.
These experiences have shown that elevated internal loading can be a significant factor in the eutrophication of lakes and coastal areas. There is a large number of unrecorded cases, but it is likely that most eutrophic lakes, to some extent, have an elevated internal load due to long-term elevated input and sedimentation of phosphorus from external sources.
A report from the Swedish University of Agricultural Sciences previously stated that the number of lakes in Sweden that have a net internal load on an annual basis is probably low. However, a low or negative net internal load on an annual basis may conceal a high gross load or a high load during the summer months with a major, negative impact on water quality. Elevated, gross internal loading is likely to be a significant contributor to eutrophication in numerous eutrophic lakes, but the amount of data available is insufficient to assess the extent of the problem.[2]
New estimates of internal loading have been made for 45 lakes as part of LIFE IP Rich Waters and a complementary project[3]. Most of the lakes surveyed had elevated internal loading. However, the magnitude of internal loading varied greatly in these lakes, and the significance for water quality and eutrophication was largely dependent on specific conditions in and outside these lakes. For instance, the internal load in Lake Övre Milsbosjön (county of Dalarna) amounted to 17.8 mg P m-2 d-1, which is very high, but the lake still has a good phosphorus status due to a strong stratification of the lake water that limits the transport of phosphorus from the bottom water to the surface water. However, when the lake water becomes mixed at the end of the summer, much of the phosphorus that diffused from the sediments can enter water bodies downstream of the lake. A much lower level of internal loading was measured in Lake Norra Lången in the county of Örebro (1–2.5 mg P m-2 d-1), but the lake there has poor ecological status. Although the internal load is considered low compared to other lakes, it represents 90 per cent or more of the phosphorus input to the lake during the summer months. By way of comparison, a typical value for internal loading in unaffected lakes is 0.25–0.5 mg P m-2 d-1.
Even if an observed, elevated internal load means that phosphorus release from the sediments is greater than in an unaffected system, the internal load needs to be related to the impact from external sources and to the phosphorus dynamics in the system in order to assess the significance of the internal load and the need for measures.
1.2 Measures against internal loading
A high external nutrient load is generally the main cause of problems with eutrophication, and so measures against eutrophication should primarily target external sources. However, external measures may sometimes prove to be insufficient, or the effect of these measures may be delayed due to an elevated internal load; which in turn is usually the result of a prolonged period of high external loading. There are measures that can be applied to reduce internal loading. Whether such measures are needed depends on the size of the internal load and how this interacts with other internal and external phosphorus fluxes in the system.
Measures to reduce internal phosphorus loading may include removing phosphorus-rich sediments from the system, binding phosphorus in the sediments by adding minerals, or oxygenating the sediments. Phosphorus can also be removed from the water by means of depletion of fish stocks or uptake of cultivated organisms (e.g. mussels), which indirectly helps to reduce the internal load. All these methods have their benefits, costs and risks – and these differ from case to case. Section 2.5 describes in greater detail a number of criteria that can be used when selecting measures to reduce internal loading.
1.3 A decision support tool with 4 steps
Most measures to reduce internal loading are costly and require extensive preparations in the form of monitoring and impact assessments. As stated, there are a large number of eutrophic lakes and coastal waters where internal loading could be a problem, and so a methodology is needed in order to effectively determine when measures need to be implemented. The decision support tool presented herein aims to facilitate such an assessment by describing a process for prioritising which water bodies should be the focus of more detailed investigations and application of measures.
2 How the decision support tool works
The decision support tool is divided into four steps, the intention being to generally apply Step 1 – which has little need for data – to all lakes in a water district. An assessment is made in this step to identify lakes at risk of elevated internal loading and which lakes should be prioritised for further investigation. Taking these lakes forward to Step 2 often requires further data collection (environmental monitoring) to be carried out in order to quantify the internal and external loading. Based on the results of Step 2, affected lakes can then be taken to Step 3 (lake modelling and scenario analysis) and possibly Step 4 (selection of measures), depending on the outcome from Step 3.
2.1 Uncertainties and applicability of the decision support tool
The uncertainty in the assessment of the impact of internal loading decreases from Step 1 to Step 3. Uncertainty is greatest when assessing risk for mixed and polymictic lakes (see section 2.2 below) in both Step 1 and Step 2. For these lake types, there is a 25–30% probability that the risk level in Step 1 is misjudged by up to two units. For dimictic lakes, the uncertainty is significantly lower for both Step 1 and Step 2. The level of uncertainty is highly dependent on the availability of sufficient data with which an assessment can be made. This is described in greater detail in Appendices 1 to 4. The risk of miscalculating the internal load using dynamic modelling in Step 3 is very low, provided that sufficient data is available. The decision support tool is based mainly on data from lakes in southern and central Sweden, and is therefore mainly applicable there. The decision support tool can also be used to assess lakes in the northern part of the country (Norrbotten, Västerbotten, Jämtland and Västernorrland), but the assessment is more uncertain. The data we have indicate that even when internal loading appears to be elevated in a lake in northern Sweden, it does not reach the same levels that may be found in eutrophic lakes in the southern part of the country, where the growing season is significantly longer than in the north. Therefore, the risk class limits may need to be adjusted for lakes in northern Sweden.
There is also uncertainty with the decision support tool if it were to be applied to coastal waters. However, the existing decision support tool and class boundaries for lakes in general are also expected to be applicable to coastal waters of the Baltic Sea in southern Sweden. When applying Step 1 to coastal waters, it is assumed that the water exchange between coastal waters and the Baltic Sea is limited.
Decision support tools optimised for coastal waters and lakes in northern Sweden are currently being developed in the project.
2.2 Step 1 – risk assessment
This step assesses whether there is a risk of a lake having an elevated internal load; that is to say, a higher internal load than if the lake were unaffected by nutrient inputs from human activity. The risk assessment differs between lake types, i.e. between shallow, fully mixed lakes and deep, stratified (dimictic) lakes. The risk assessment scale (table 1 and table 2) was developed using quantitative data on the internal load of a large number of lakes; mainly in Sweden, but in other countries as well. The data needed and approach for applying the decision support tool are described in detail in Appendix 1. The type of lake can be assessed using temperature and oxygen profiles in the water column during the summer months, the geomorphological characteristics of the lake, or simply the bottom water temperature when taking samples in late summer. This is described in greater detail in Appendix 2.
A high risk means that the internal load is probably elevated and can be expected to significantly increase the amount of phosphorus in the lake water and thereby contribute to eutrophication. However, there may be exceptions where a high risk does not lead to a correspondingly high contribution to eutrophication. For instance, strong stratification of the water column in a lake may prevent phosphorus from being transported to surface water, except when the lake is mixed in spring and autumn. Another example is a lake where the deepest point with a high internal load represent a small fraction of the total lake area, so that the elevated internal load has a relatively low contribution to the combined external and internal load.
If the risk is deemed to be low, it is likely that the internal load is not elevated and the contribution from the internal load is negligible.
In the case of shallow lakes that are fully mixed throughout the summer, the risk assessment is based on the percentage increase in the phosphorus concentration in the lake water between the lowest measured phosphorus concentration in spring (February–June) and the highest concentration in late summer or early autumn (August–September). This assessment is made on a 4-point scale (table 1).
It is important to be aware that an increase in lake phosphorus concentrations may be due to an increase in external loading, as well as internal loading during the summer months. If it is suspected that the former may be the case, the load from external sources needs to be investigated further: this is done in Step 2.
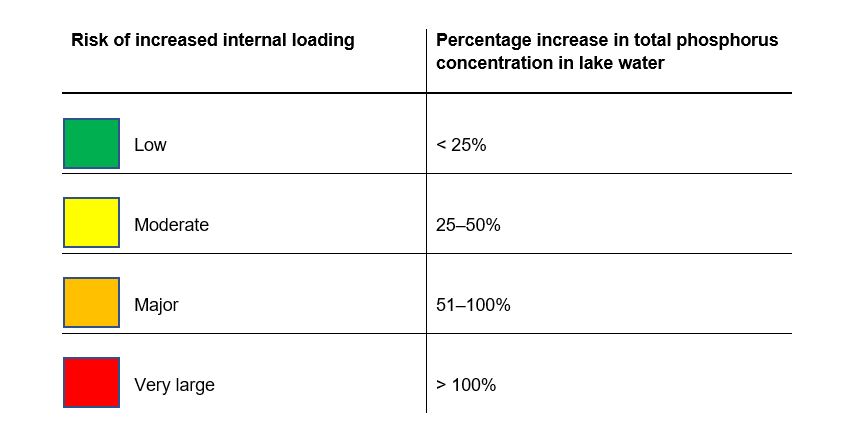
In the case of deep, dimictic lakes with stable stratification throughout the summer period, the risk is assessed on a 5-point scale solely on basis of the phosphorus concentration in the bottom waters of the lake at the lake’s deepest point(Table 2). The risk assessment for dimictic lakes is fairly certain as stratification remains stable throughout the summer in these lakes, and any impact from external sources mainly affects phosphorus concentrations in surface waters, while concentrations in the water below the thermocline remain relatively unaffected.
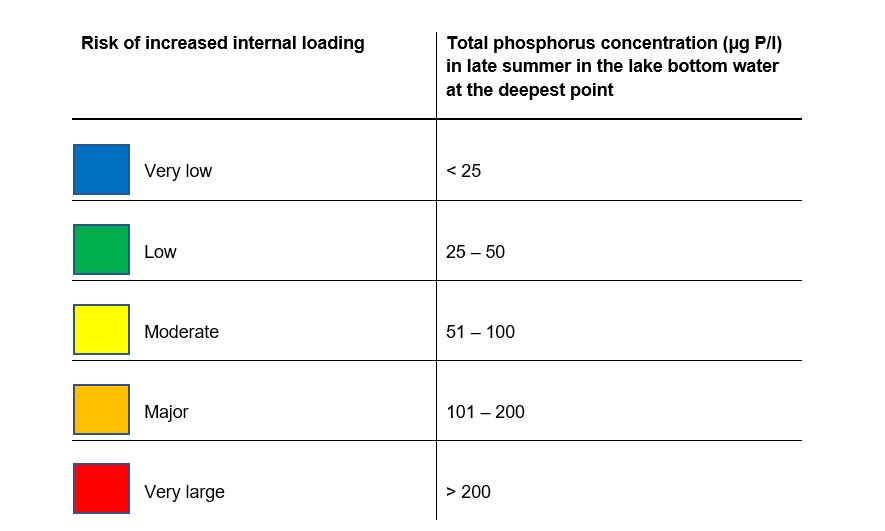
If the lake is covered with ice for a fairly long, continuous period of at least 4 months, the risk assessment for internal loading can also be based on the phosphorus concentration of the bottom water just before the ice breaks up. The risk assessment scale in table 2 is then used for all lake types. However, the risk can be expected to be underestimated using the scale in table 2 as the water temperature is lower in winter. Further work with data from ice covered lakes is required in order to develop this decision support tool further. Polymictic lakes have unstable stratification and are neither fully dimictic nor fully mixed throughout the summer. Stratification may occur in such lakes during calm periods in the summer, but this is broken when the weather becomes windier, for example. Phosphorus from internal loading may then accumulate in the bottom water while the water is stratified, but is then distributed throughout the water column when the stratification is broken. The risk assessment for such lakes must be performed using both the mixed and dimictic lakes method. This is described in greater detail in Appendix 1. The assessment of risk for polymictic lakes is always uncertain as the lake’s point on a scale between mixed and dimictic conditions is unknown at the time of sampling in late summer. The conditions are neither fully dimictic nor mixed, so the risk assessment of polymictic lakes is often an underestimate of the real risk.
Four years of data should be used for all lake types in order to maximise the reliability of the risk assessment. The risk is then assessed for each year, and a mean is calculated. Internal loading as a source of influence can be dismissed if the mean indicates that the risk is low or very low. More measurements may be needed if the mean indicates moderate risk with a certain amount of variation between years. For dimictic lakes, it is also possible to take into account the proportion of the lake’s area that is stratified (i.e. the lake area with depths below the thermocline). If this is less than about 10% and the risk is moderate, the internal load does not normally make a significant contribution to the amount of phosphorus in the lake water, and proceeding with Step 2 is probably not justified. However, if the risk is deemed to be high or very high, one should proceed to Step 2 in order to quantify the internal load.
2.3 Step 2 – environmental monitoring and quantification
Step 2 quantifies the internal load of the lake in units of kg P and quantifies the internal loading rate, Li, expressed in mg P m-2 d-1). The internal loading rate is used to assess whether internal loading is elevated or not. The internal load expressed in kg P can be compared with the external load over the same period. To quantify the internal load, more measurements are normally required than the usual environmental monitoring carried out to assess the ecological status of a lake. The data needed and approach for calculating the internal load as described in Step 2 are explained in detail in Appendices 3 and 4.
As in Step 1, a distinction is made between dimictic, polymictic and fully mixed lakes in the data needed and how the internal load is calculated. Data series for at least two years are needed for all lake types as there may be significant differences between years depending on summer weather conditions.
In shallow, fully mixed lakes, phosphorus concentrations in the lake’s surface water, for example, are measured at least monthly, from early spring (after thawing) to early autumn (usually September or October). Changes in the amount of phosphorus in the lake water can be calculated using lake water volume data. An increase in amounts of phosphorus in lake water during the summer months is often the result of internal loading. However, phosphorus inputs and outputs must be taken into account in order to calculate the contribution from internal loading using a mass balance approach. Monthly sampling and measurement of phosphorus concentrations and water flow in the largest inflows and outflows is therefore needed. As the internal load is calculated from a mass balance for phosphorus, it is sensitive to correct measurements of inflows and outflows, especially when the turnover time of the lake is short. Figure 2 shows an example of a lake with very low inflow and outflow during the summer months (mean annual turnover time of 0.31 year[4]) where the internal load completely drives the increase of the amount of phosphorus in the lake water.
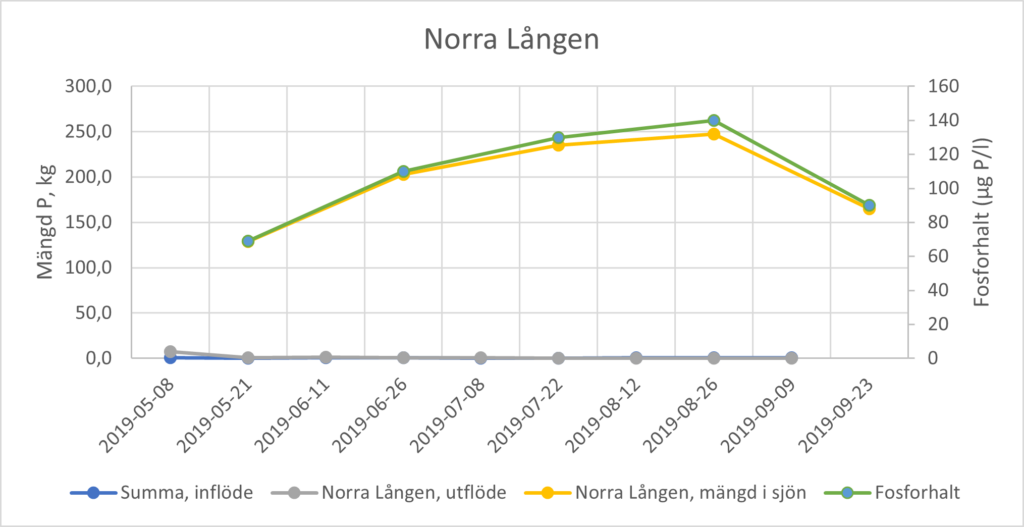
In dimictic lakes, profile data for total phosphorus concentrations in the water column are used to detect and quantify internal loading. Phosphorus released from the sediments accumulates below the thermocline, leading to a typical phosphorus gradient from the bottom of the lake up to the thermocline (Figure 3). The internal load is quantified by multiplying the phosphorus concentration by the water volume for each depth interval. Interpolation between sampling depths takes place for the concentration in order to calculate the phosphorus volume for each depth level. To calculate the internal load reliably, monthly sampling is needed from the time of spring mixing (usually between March and May) to autumn mixing (usually between September and November). Sampling takes place at the deepest point of the lake.
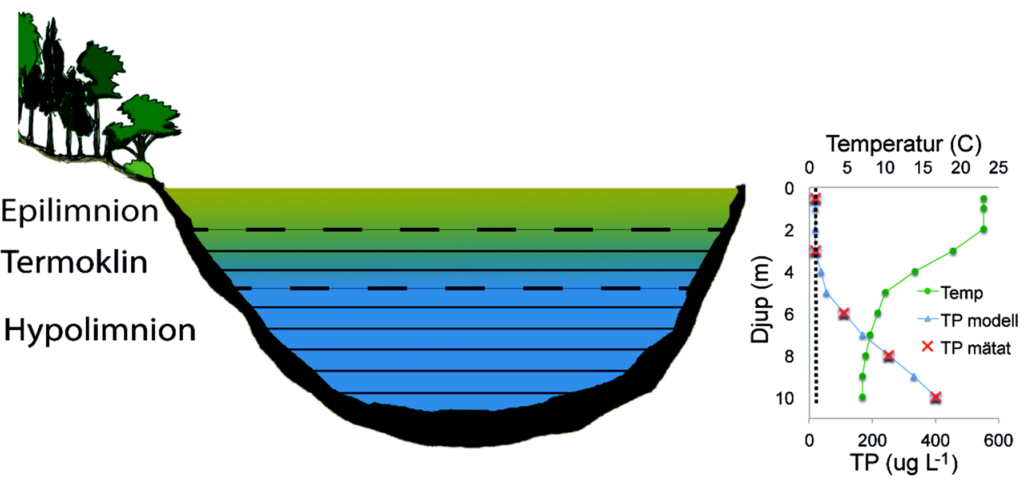
In the case of polymictic lakes with unstable stratification, data need to be collected according to the protocol for fully mixed lakes and that for dimictic lakes. The calculation of the internal load is based on a mass balance for phosphorus as for a fully mixed lake. However, the main difference here is that changes in the amount of phosphorus throughout the entire water column are calculated using the phosphorus profile (the same as a dimictic lake), and not just the phosphorus content of the surface water. The internal load is calculated as the increase in the amount of phosphorus in the entire lake between the minimum amount in spring and the maximum amount (the same as for a fully mixed lake), which is often in late summer. As for fully mixed lakes, the calculation needs to take into account external phosphorus inputs and outputs.
Phosphorus turnover in fully mixed lakes and polymictic lakes with unstable stratification is often highly dynamic. Sampling more frequently than once a month may be necessary in these cases. This does not always resolve the issue, however, as there may be periods when internal loading dominates and the amount of phosphorus in the water increases, and other periods when phosphorus sedimentation dominates (negative internal loading) and the amount of phosphorus in the water decreases. In such lakes, dynamic modelling is usually needed in order to model and calculate sedimentation (a sink) and internal loading (a source) separately.
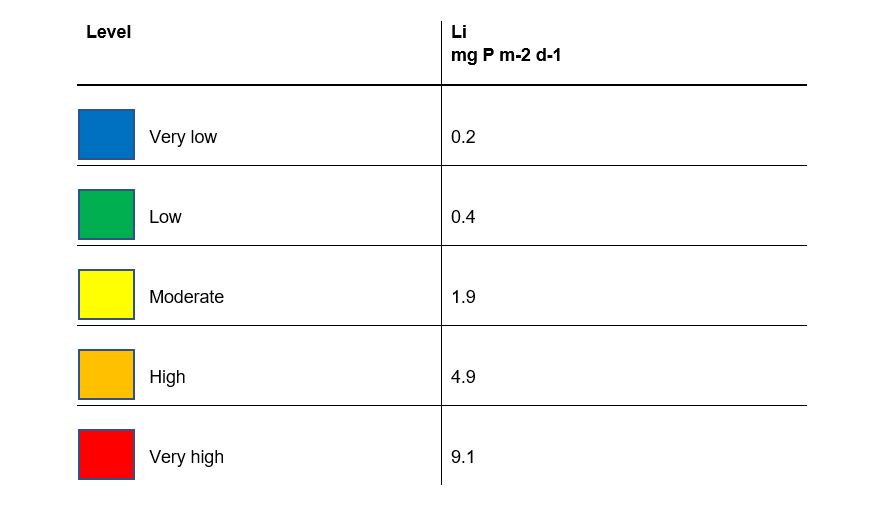
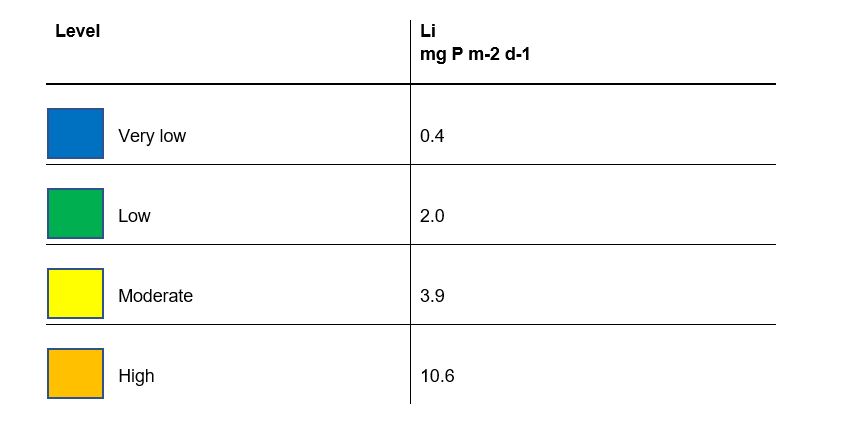
The calculated mean rate of internal loading Li (expressed in mg P m‑2 d‑1) can be compared with the rates in unaffected lakes in order to assess the risk of an elevated internal loading rate. This is done using Table 3 for mixed lakes and Table 4 for dimictic lakes. The highest risk level exceeded in a season with respect to Li is used to assess the lake. It may be motivated to proceed to Step 3 if the level of the internal loading rate is moderately elevated or more. When assessing whether it is motivated to proceed to Step 3, the decision may also take into account the magnitude of the estimated internal load (in kg P) relative to the external load during the same period. The ecological status of the water body with regard to the nutrient parameter may also be considered. If the internal load is high but the status is good or higher (which is possible in highly stratified lakes), Step 3 can nevertheless still be considered if there is a risk that downstream water bodies are affected by the increased phosphorus load from the sediment when the lake water column mixes and excess phosphorus can be transported downstream.
Moving directly from Step 2 to Step 4 (selection of measures) may be considered in individual cases. For instance, the costs for a detailed study of phosphorus dynamics may not be motivated for smaller lakes where the cost of action is relatively low. However, this assumes that the results from Step 2 clearly show that the lake is affected by elevated internal loading that is significant relative to external phosphorus loads, and that it contributes significantly to eutrophication issues in the lake. Lake Lången (see Figure 2) may be regarded as such an example.
2.4 Step 3 – modelling
Modelling allows the phosphorus dynamics of an aquatic system to be investigated in greater detail, and the effect of different intervention strategies can be simulated in a scenario analysis. This means that it is possible to make a trade-off between the benefits of measures against external inputs and measures against internal loading. Simulation models can also be used to assess the longevity of measures against internal and external loading. Modelling can also be used to simulate how many years it would take for natural recovery to reduce the elevated internal loading to background levels.
For satisfactory investigation of how a lake or coastal water will respond to a certain measure, or how different measures can be combined to achieve a desirable improvement in water quality, an adequate simulation model needs to be used. It is important for the model used to be based on mass balance, and for it to handle both external flows (inflows and outflows) and the internal fluxes shown in Figure 1. The model also needs to be able to cope with seasonal dynamics. More specifically, the model has to deal with phosphorus inflows to the system via watercourses and any point sources. It must also take into account the water turnover (hydrology) at different times of the year in order to calculate the amount of phosphorus flowing out of the system. A number of internal processes such as water exchange between surface and deep water, sedimentation, resuspension and diffusion from the sediments also need to be taken into account. Resuspension and diffusion are the processes that generally contribute to internal loading. The importance of these processes depends on in-lake conditions that affect phosphorus mobility in the lake in different ways. The importance of these processes also depends on how large the supply is in relation to other phosphorus inputs. That is why it is particularly important to ensure that the calculation of resuspension and diffusion is included in the model in order to simulate measures against internal loading. One example of a model that fulfils these requirements is the LEEDS model, which has been used to simulate phosphorus dynamics and measures in a number of Swedish lakes and coastal areas.[5],[6],[7] Such modelling requires special expertise.
Criteria for useful simulation models in Step 3:
- Based on mass balance
- Includes all fluxes indicated in Figure 1.1
- Includes water turnover in the lake and water exchange between surface water and deep water
- Models seasonal dynamics
There are also more comprehensive simulation models that explicitly include biological processes, allowing parameters such as water transparency, nitrogen, and phytoplankton populations to be predicted. The emphasis in this guide is, however, solely on phosphorus, as there is a close relationship between phosphorus levels in a lake and the presence of important phytoplankton groups that contribute to algal blooms[8]. If a more complex relationship is suspected between phosphorus or other nutrients and important biological parameters, models including biological processes may be justified.
Lake Hjälmaren provides an example of how a modelling study of measures against internal loading can be performed. This study used the LEEDS model to evaluate the impact of different combinations of measures in the four basins of the lake. In this scenario analysis, an evaluation was made of the expected reduction in the mean annual phosphorus concentration of the lake’s surface water in response to a 50% reduction in inputs from external sources (rivers and point sources), a 50% reduction in internal loading in the lake’s central basin (Storhjälmaren), and a combination of these two measures (see Figure 4).
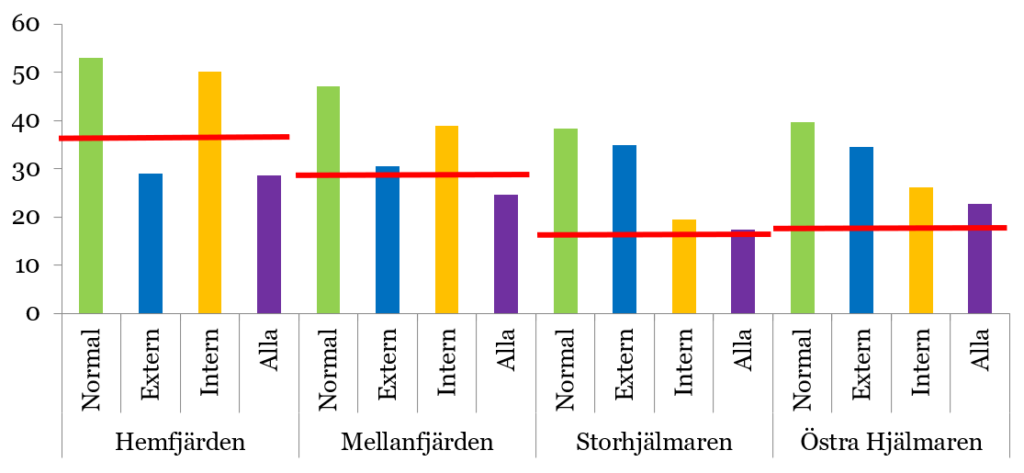
One important observation is that the model predicts that the lake’s four basins respond quite differently to the measures tested. Reduced external input has a major effect on phosphorus concentrations in two basins, Hemfjärden and Mellanfjärden, and, according to the model, would be sufficient to achieve good status in these basins. However, the effect of reduced external input is limited in the two other basins, Storhjälmaren and Östra Hjälmaren, while reduced internal loading appears to have a major effect. The fact that the effect of reduced internal loading according to the model is greatest in Storhjälmaren is expected, as this is where the measure is applied. Overall, this modelling shows that the design of measures has a significant impact on performance and that each system has unique conditions, even within the same lake. That is why it is advisable to carry out dynamic mass balance modelling according to the principles described here before committing economic resources to the implementation of measures. More detailed information on the modelling of Lake Hjälmaren is described in Malmaeus & Karlsson (2015).[9]
2.5 Step 4 – selection of measures
2.5.1 Possible measures
This chapter outlines possible measures against internal phosphorus loading. These measures are divided into four categories: permanent fixation, removal, biomanipulation and oxygenation and mixing (Table 5). See Part II “Detailed description of measures” for a detailed description of the measures. Measures deemed to be under development are referred to briefly here, but are not included in Part II.
Method | Applications |
Permanent fixation | Aluminium precipitation, addition of clay with added phosphorus-binding substances |
Removal | Conventional dredging, low flow dredging, bottom water withdrawal |
Biomanipulation | Reduction fishing |
Oxygenation | Mixing, aeration |
2.5.1.1 Permanent fixation
Permanent fixation means that phosphorus is bound in the sediments and then permanently buried. This requires addition of phosphorus-binding substances that are effective under the conditions prevailing in the water body and the sediments. This means, for example, that they have to be able to bind phosphorus regardless of redox (oxygen) conditions.
2.5.1.1.1 Aluminium precipitation
Aluminium precipitation can take place in the water body (water treatment) or directly in the sediment (sediment treatment). In the case of water treatment, dissolved phosphorus is precipitated by adding an aluminium salt solution to the water body, which causes an aluminium mineral to form, followed by the settling of the mineral particles. In sediment treatment, the aluminium salt solution is mixed with lake water to form the aluminium mineral, and the mineral is then injected directly into the sediment, where it binds to phosphorus. This measure reduces the amount of phosphorus that can diffuse into the water body, and thus reduces internal loading. The pH of the above-mentioned sediment surface needs to be in the 6–8.5 range to avoid toxic effects of aluminium on biota.
Phosphorus fixation using aluminium is a well-documented method for treating freshwater lakes.[10] The 2012–2013 treatment of Björnöfjärden Bay in the municipality of Värmdö was the first time the measure was tested in brackish water.[11]
2.5.1.1.2 Phosphorus-binding substances in bentonite clay
In this method, clay with added phosphorus-binding substances such as aluminium or lanthanum is added to the lake by dispersal in the water column. The clay sinks through the water, and mobile phosphorus is permanently bound in the sediment. Full-scale tests using a mixture of bentonite clay and lanthanum (PhoslockTM) were conducted for the first time in 2001[12];[13] and more experiments have been carried out since then using lanthanum, aluminium and calcium in different clays.
2.5.1.1.3 Other phosphorus-binding materials
Materials under development include reactive filter materials made from opoka rock, which is made up of calcium carbonate and silicon (Polonite). Opoka has a high porosity and surface area where sorption can take place. The binding of phosphorus is long-term.[14]
Another material that can be used is made of granulated modified zeolite, which occurs naturally as a mineral of aluminium silicates but can also be produced synthetically. This material is porous, and therefore has a large reactive surface area. There is also zeolite that has been modified with aluminium salt to enhance its capacity to bind phosphorus. The granulate has been proven to retain both nitrogen and phosphorus, but needs to be studied in full-scale experiments before its potential as a restoration method can be evaluated.[15]
Marl treatment is another method under development. This involves enhancing the fixation of phosphorus in sediments by adding marl (calcareous clay), which adsorbs phosphate. Marl is a particulate residue from the extraction of limestone and contains large amounts of calcium carbonate. Granules of marl can be released into the water, and dissolved phosphate is fixed on the surface of the marl particles by adsorption as they make their way to the bottom. Laboratory and pilot studies have shown that adsorption of phosphorus to marl can occur even at low temperatures and under low oxygen conditions.[16]
2.5.1.2 Removal of phosphorus
Removal means the direct removal of phosphorus from the water body. This can be done either by withdrawing phosphorus-rich water from the lake or by dredging phosphorus-rich sediment.
2.5.1.2.1 Hypolimnetic withdrawal
Hypolimnetic withdrawal involves withdrawal of deep lake water containing high concentrations of phosphorus. This method is best suited to stratified lakes and can be a relatively inexpensive alternative for addressing internal loading. However, this assumes that extensive temperature changes in the hypolimnion are avoided, and that the water withdrawn is disposed of in a way that does not adversely affect downstream water bodies. This method has been used in a number of lakes, mainly in Central Europe, since the mid-20th century.[17],[18]
2.5.1.2.2 Dredging
Dredging involves the removal of sediment from water body. By removing the upper most nutrient-rich layer of sediment, release of phosphorus from lake sediments may be reduced. The measure is described only briefly in this guide, as detailed criteria for dredging are addressed in the Swedish Agency for Marine and Water Management’s guide on dredging and management of dredged material.[19]
Low-flow dredging of lake bed sediments is a measure under development that is being tested in a number of restoration projects in various lakes in Sweden.[20],[21],[22] This method is a gentler form of dredging that involves carefully sucking up surficial sediment (only the upper few cms) in hoses in order to minimise particle dispersal. One challenge presented by this method is the handling of the sludge, which has a high water content. After dredging, the removed nutrient-rich sediment has to be dewatered and the return water treated to remove phosphorus and any contaminants. The nutrients in the dewatered sediment can be used as fertiliser in agriculture and forestry if the sediment does not contain excessively high levels of pollutants. If there is an opportunity to dispose the sediment locally, this is preferable, as it minimises the need for transport thereby reducing the environmental impact.
2.5.1.3 Biomanipulation and reduction fishing
Biomanipulation attempts to alter the structure of an ecosystem by selectively reducing and/or increasing the number of individuals within certain species groups. This sometimes makes it possible to achieve desired changes in the ecosystem that are beyond those occurring as a direct effect of the reduced amounts of phosphorus in the lake system brought about by the measure. The comparison between measures in Table 7 below does not take into account this effect of biomanipulation. Biomanipulation as a measure for managing internal loading can be similar to reduction fishing. Reduction fishing aims to increase the predation pressure on phytoplankton by removing fish species that consume zooplankton, and to reduce the release of phosphorus from the sediment through bioturbation. [23] Reduction fishing per se also contributes to significant removal of phosphorus from the system. The method is a common measure applied in eutrophic lakes.[24] The target for reduction fishing is usually what is known as white fish, i.e. cyprinids such as roach and bream.
2.5.1.4 Oxygenation and mixing
As their names suggest, oxygenation and mixing aim to increase oxygen in the parts of the water body that are at risk of oxygen deficiency. One of the desired effects of these methods is to reduce the release of phosphorus that may occur at low redox potential (i.e. low oxygen conditions) from sediments containing iron-bound phosphorus.
2.5.1.4.1 Mixing of lake water
This method involves mixing the water column to oxygenate the water throughout the lake, thereby increasing the oxygen concentration in the bottom water. The aim of this is to increase the retention of iron-bound phosphorus in the sediment. Mixing may, however, cause increased primary production of phytoplankton and there are no examples that show that the method has been successful. The method and the underlying principle have been questioned in recent decades due to the general lack of success.
2.5.1.4.2 Oxygenation and aeration of the hypolimnion
This method involves adding oxygen to deoxygenated bottom waters and has been used in lakes since the mid-20th century. The purpose of the measure is to oxygenate the bottom water so that the sediment can thus bind phosphorus at the sediment surface. This measure is implemented by allowing bottom water to come into contact with oxygen or air in various ways.
2.5.2 Environmental impact of measures
Life cycle assessments (LCAs) can be used to evaluate the effectiveness of measures against internal loading. Their overall environmental impact is considered, taking into account not only the positive effect of the measures in mitigating eutrophication symptoms, but also their potentially adverse environmental impact in terms of, for example, carbon dioxide emissions (Figure 5).
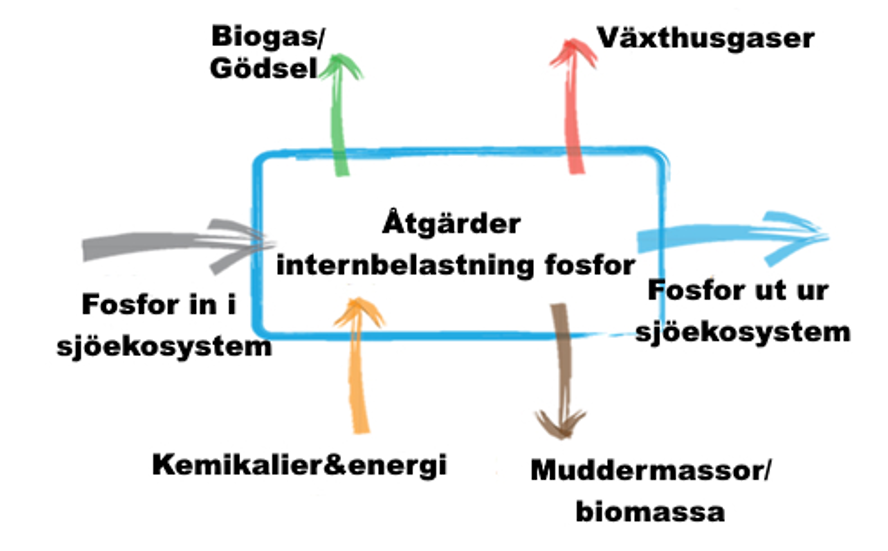
LCA is essentially a method for the holistic elucidation and assessment of the environmental aspects and potential environmental impacts associated with a product or system (ISO 14040:2006 and 14044:2006). Assessment is performed by identifying and collating relevant inflows and outflows of the system, evaluating potential environmental impacts associated with these flows and interpreting the results. A full LCA covers the entire life cycle of the system studied, from extraction of raw materials, processing of raw materials and energy, manufacturing processes, transport, use and recycling to final disposal. Resource use, human health and ecological impacts are the general categories of environmental effects normally considered.
In a simplified LCA carried out at IVL Swedish Environmental Research Institute, emphasis was placed on comparing the climate impact of different measures used to reduce internal loading. Table 6 lists a number of different measures and the main processes within their life cycle giving rise to carbon dioxide emissions. The underlying assumptions and results at a more detailed level are presented in Appendix 6, “Carbon footprint of various measures”.
Measure | Causes of carbon dioxide emissions | Causes of carbon dioxide emissions |
Aluminium precipitation | Production, transport and distribution of precipitation solutions | 60 |
Conventional dredging | Collection, transport, dewatering and disposal of dredged material | 25 |
Low-flow dredging | As above for conventional dredging, but to a lesser extent | 12 |
Reduction fishing | Is more of a potential carbon sink if the catch can be utilised as energy, animal feed or food | -24 |
Of course, it is difficult to draw general conclusions about the carbon footprint to which various measures give rise, as this is influenced by the site-specific conditions where the measures are implemented. The levels presented in Table 6 should therefore not be taken literally when making decisions on intervention strategy. However, they do illustrate an important principle: different measures can result in widely varying amounts of greenhouse gas emissions for the same amount of phosphorus removed. Implementation of a measure per se can also reduce greenhouse gas emissions from lakes by reducing eutrophication. However, there are not enough data available to quantify this, or to indicate whether there is any difference between different types of measures. It may therefore be advisable in individual cases to carry out a simplified LCA in which the carbon footprint and other potential environmental impact(s) of the measures under consideration are compared and used as a basis for the choice of intervention strategy.
2.5.3 Social and economic aspects of intervention work
2.5.3.1 Social and economic effects
The implementation of measures to reduce internal loading may also have significant social and economic effects. Identifying these effects can generally lead to a greater understanding and acceptance of and motivation for often costly measures. Direct economic benefits may include savings, increased employment and new business opportunities. There are also potential socio-economic benefits by means of social improvements, such as enhanced well-being.
The LIFE IP Rich Waters project evaluated the social and economic effects of aluminium treatment in Lake Norrviken.[25] This evaluation shows that the treatment has provided the municipalities of Sollentuna and Upplands Väsby with an opportunity to highlight their environmental commitment and to communicate on water management in a wider perspective. Residents’ knowledge of and commitment to the lake also increased. The project conducted a survey asking residents whether they perceived any difference in water quality. In their responses, many people said that the lake water was clearer and of better quality than it had been for a very long time. The clearer water is considered to be more attractive, and the value of the lake for recreation and swimming has increased as a result.
Individual measures for lakes and watercourses may seem cost-intensive, but improving water quality, particularly in recreational lakes close to densely populated areas, can provide significant socio-economic value. A valuation study conducted in Stockholm showed that the socio-economic benefits of achieving good water status in all watercourses in the municipality were estimated at SEK 2.5–2.8 billion, while the costs of the measures required to achieve this were estimated at around SEK 1 billion.[26] It is difficult to perform a value transfer (use a result for one area to perform an estimate in another) to Norrviken on the basis of the study. However, we know from the study that lakes used for swimming and recreation often yield high values in terms of socio-economic benefits for improvement of water quality. That is why it is likely that the value of improving water quality in Norrviken should also be estimated to be relatively high.
2.5.3.2 Cost of the measures
The costs of implementing measures to manage internal loading of course vary from case to case, and also with time as the different technologies are further developed. Table 7 provides some standardised costs based on calculations reported in previous studies[27],[28], supplemented by some new data. The costs are expressed as per kg of phosphorus removed from the system or made immobile as a result of the measure.
Measure | Cost (SEK/kg separated P) |
Aluminium precipitation | 400–700 |
Conventional dredging | 1000* |
Reduction fishing | 750**–2 000 |
Low-flow dredging | 70,000–80,000*** |
2.5.3.3 Funding opportunities – LOVA grants
In the case of internal phosphorus loading, it may be impossible to identify and determine who is responsible for taking action against the damage caused. Actions against internal loading are therefore often carried out on a voluntary basis. In Sweden, voluntary measures against internal loading can be funded by government grants, such as annual LOVA grants or via specific targeted calls.
The purpose of the LOVA grant is to help achieve the environmental quality objectives set for the aquatic environment, including measures against eutrophication and internal loading. It is mainly available to municipalities and non-profit organisations such as water conservation associations. The LOVA grant is regulated by the Ordinance on state funding for local water management projects (2009:381). The Swedish Agency for Marine and Water Management is authorised to issue more detailed regulations on matters such as eligible measures and what is required of an application.[29] See www.havochvatten.se for further information.
2.5.3.4 Special initiatives
In some cases, the Swedish Agency for Marine and Water Management can implement special initiatives and targeted calls within priority subject areas. Public stakeholders and non-profit organisations can apply for such grants. Information about special calls is provided on the Swedish Agency for Marine and Water Management website at www.havochvatten.se/anslag-bidrag-och-utlysningar when such calls take place.
2.5.4 Summary of the measures
Finally, Table 8 provides an overall assessment on a relative scale of the applicability, costs and carbon footprint of a number of different measures.
Measure | Applicability | Disadvantages/ uncertainties | Potential positive side effects | Cost | Carbon footprint |
Aluminium precipitation | General, well tested | Energy and resource-intensive | Low | High | |
Conventional dredging | Smaller areas | Energy and resource-intensive | Medium | High | |
Low-flow dredging | Under development | Nutrient utilisation on arable/forest land | High | Medium | |
Hypolimnetic withdrawal | Stratified water bodies | Potential relocation of problems downstream | Nutrient utilisation on adjacent arable/forest land | ||
Oxygenation | Hypoxic areas | Need to continue for a long time | Beneficial for higher wildlife | Low | Medium |
Reduction fishing | Areas with high densities of white fish | Potential removal of food resource for predatory fish | Beneficial for lake flora and fauna (biomanipulation), utilisation of food/feed resource | Medium | Small |
Part 2. A detailed description of measures
Authors:
Robert Almstrand, Swedish Agency for Marine and Water Management, Gothenburg
Brian Huser, Swedish University of Agricultural Sciences, Uppsala
Magnus Karlsson, IVL, Swedish Environmental Research Institute, Stockholm
Demarcation
Part II of the handbook covers measures in inland lakes to prevent the diffusion of phosphorus from sediments and the direct removal of sediments and nutrient-rich bottom water. It does not cover measures in coastal and marine areas.
Part II of the handbook is not intended for use as a manual for implementing measures. It should be regarded as an aid when assessing whether an internal loading measure may be appropriate and can provide information on the advantages and disadvantages of various intervention techniques. A careful analysis needs to be conducted in each case, however, in order to compare potential measures with one another on the basis of site-specific conditions.
1 Aluminium precipitation
Aluminium precipitation involves the precipitation of dissolved phosphorus by adding an aluminium salt solution to the water body (water treatment) or injecting it into the sediment (sediment treatment). The phosphorus is then fixed in the sediment, which reduces the amount of phosphorus that can diffuse into the water body and thus the internal load. This method uses the same principle as at drinking water treatment plants, where aluminium salt is added to precipitate unwanted substances out of the water. Phosphorus fixation using aluminium is a well-documented method for treating lakes and has been used since the 1970s.
Method description
There are two main methods for binding phosphorus with aluminium. Either an aluminium salt solution is distributed in the water column, or the solution is mixed with lake water and harrowed into the sediment to a depth of 10-15 cm [1]. When aluminium salt is added to the water, an aluminium mineral is formed that then settles to the sediment. Experience suggests that other substances along with phosphorus, such as various metals, may also be precipitated and removed from the water column during aluminium addition. The mineral then settles on the sediment where phosphorus in the sediment is permanently inactivated. This is the main method of phosphorus reduction even though some phosphorus in the water column will also be removed via binding to the mineral.
If the aluminium mineral is injected directly into the sediment instead, it comes into direct contact with higher concentrations of phosphorus at depth in the sediment. The resulting aluminium mineral then remains in the sediment regardless of which method is used. The main advantages of sediment treatment are that mineral location within the sediment is more controlled than with water treatment, and the binding efficiency can be improved. The dosage can be adapted here depending on the amount of releasable phosphorus in the sediment, and be adjusted for bottom areas containing differing amounts of sediment phosphorus. The cost is higher, however, if binding efficiency improves enough compared to a water treatment, the cost difference may be negligeable in some cases.
The ability of aluminium to bind phosphorus in stable mineral compounds means that this treatment is able to fix phosphorus in the bottom sediments and that oxygen deficiency no longer leads to the release of phosphorus into the water phase. The external phosphorus input, the pH of the water, the dosage of aluminium, the size of the catchment area in relation to the area of the lake and the morphology and morphometry of the lake are some of the most important factors determining the long-lasting effect of aluminium treatment [2, 3]. In general, mobile phosphorus is bound at a weight ratio of 5–10 to 1, i.e. 5–10 grams of aluminium binds 1 gram of phosphorus [4, 5, 6]. Methods to determine the optimal dosage of aluminium have evolved over the last few decades. Nowadays, it is possible to adjust the dosage on the basis of the total mobile (releasable) phosphorus content of the sediment [7]. Another option involves what is known as dynamic dosing, which is based on the amount of mobile phosphorus that needs to be bound in order to reduce internal loading to an acceptable level. The phosphorus binding capacity of aluminium, however, has differed greatly in previously treated lakes. To optimise the binding efficiency and avoid addition of excessive amounts of aluminium, an aluminium treatment may have to be split with smaller doses being added over a number of years, rather than adding the entire dose all at once. See [8] for a method for calculating whether dividing the dose is necessary.
Location
Aluminium treatments in deep, stratified lakes have generally been more successful than in shallow lakes with low buffering capacity (alkalinity), but there are exceptions to this. For instance, successful sediment treatments have been carried out even in shallow, unstratified lakes [1]. High abundance of benthic fish species (e.g., cyprinids) increases the amount of releasable phosphorus available and the sediment mixing depth needs to be increased in such cases [9]. This is because fish bioturbate, i.e., stir up sediment, when they dig for food in the sediment [9, 10]. The aluminium dose may then need to be adjusted to account for this.
The solubility of aluminium in water is dependent on pH, and the pH should be between 6 and 7 during treatment in order to maximise the binding of phosphorus and minimise the risks associated with elevated aluminium levels in the aquatic environment. The risks to benthic fauna, plankton and fish are usually considered low in waters where the pH is between 6 and 8.5 [11]. This is because aluminium has low solubility or is bound to organic matter in this pH range, which means that it has low reactivity and hence low bioavailability as well. The risks associated with aluminium toxicity increase dramatically outside this pH range (see the section entitled “Disadvantages and risks” below). Note that the measure may be inappropriate even if the pH is within an appropriate range, such as in more sensitive aquatic environments with low buffering capacity [12].
Timing
Often spring and autumn periods, when most lakes are well mixed, are most suitable when using the water treatment method because the mobile forms of phosphorus are in the sediment where it can be bound by the aluminium mineral. Appropriate timing of treatment does, however, vary depending on site-specific conditions, as well as the aluminium addition method chosen, and should therefore be considered in each individual case. Treatment should always be planned so as to avoid resuspension and transport of the flocculated material. Summer is usually avoided if the treatment is to take place in the water body, because algae in the water can interfere with the precipitation of the mineral. Late summer – i.e., before autumn mixing – may be a suitable period for sediment treatment in stratified lakes. However, before the lakes Växjösjön and Södra Bergundasjön were treated, it was found that autumn could be the most appropriate time as the local water chemistry conditions are good at that time, while there is less risk of negative effects on biota [13].
Disadvantages and risks
Aluminium precipitation has been used for decades and the technology has been the subject of extensive research and major risks of the method have been studied thoroughly. The risks from aluminium precipitation are mainly associated with the soluble forms of aluminium under acidic (pH <6) and basic (pH >8.5) conditions. Aluminium toxicity to fish and benthic fauna is one of the most serious effects of acidification of the aquatic environment where pH of lake water in Sweden reached 4.5 in some cases.
For lakes with low buffering capacity, adding aluminium to the water body can in some cases lead to undesirable changes in pH, as hydrogen ions are released when aluminium is hydrolysed in the water during the formation of the aluminium mineral. This can be avoided by means of a dosage that takes into account the alkalinity of the water [14]. Undesirable changes in pH can also be avoided to an extent by using buffered forms of aluminium, dividing the dose into several parts that are administered at different times, or adding lime (e.g. limestone flour) can be added prior to treatment.
Even though the ecological risks of aluminium precipitation performed properly have been deemed to be low in a pH range of between 6 and 8.5. Continuous exposure to aluminium has been shown to have greater adverse effects than single treatments, but continuous, long-term aluminium addition for reducing internal phosphorus loading from sediment is not a method recommended in this document [11].
The solubility of aluminium increases with decreasing pH (<6). Dissolved aluminium ions can then precipitate in environments where the pH is higher than in the surrounding water, such as on the gills of fish. The fish’s oxygen uptake is reduced as a result, and the ion balance in the blood is adversely affected [15]. Aluminium toxicity to fish and benthic fauna is regarded as one of the most serious consequences of acidification of the aquatic environment. However, there is unlikely to be a great risk of aluminium toxicity in lakes treated with aluminium as long as treating lakes with low alkalinity (buffering capacity) is avoided. Natural buffering processes can maintain a significantly higher pH in the sediment compared to the water body and the sediment surface [16]. Even if not injected into the sediment, the precipitated aluminium is buried in the sediment a number of years after treatment, and is no more sensitive to the effects of low or high pH than the aluminium already present naturally in the sediment.
Aluminate (Al(OH)4–) is formed at high pH (>8.5). Aluminate was not regarded as toxic previously, but empirical data from the lake Vedsted Sø in Denmark and subsequent laboratory experiments have shown that aluminate is toxic in water with low buffer capacity [12]. Moreover, studies [17] show that the stability of the bond between aluminium and phosphorus is adversely affected at pH 8 or higher, and the measure becomes less effective. Elevated pH in lakes occurs mainly when carbon dioxide is absorbed in large quantities by phytoplankton during photosynthesis, usually as a result of eutrophication. The pH can be very high (pH >9) in shallow (polymictic) and highly eutrophic lakes and bays. If there is a risk of such conditions occurring, it is more appropriate to carry out the treatment at a time when conditions are more favourable [18], or to use another measure. However, the pH in deeper lakes may be significantly lower in the bottom water, and sediment treatment may then be an option even if pH >8.5 in the surface water.
Biota may be exposed to elevated levels of aluminium during the treatment. In general, eggs and larvae, i.e. younger life stages, are more sensitive than adults, mainly because they cannot move away from the exposed area. The degree of exposure and impact are dependent on the aluminium precipitation method chosen, but also varies between species. For instance, a study on the level of accumulated aluminium in fish gills after aluminium precipitation in the hypolimnion [19] did not detect elevated levels in roach, bream or silver carp. That said, elevated levels of aluminium were observed on the gills of perch. A comparison of sensitivity to aluminium between Scandinavian fish species found that salmon, roach and minnow were the three most sensitive species, followed by perch, grayling, brown trout and Arctic char [20].
In Björnöfjärden, where aluminium was injected directly into the sediment, elevated levels of aluminium were observed in water and biota during and after the aluminium treatment. However, the levels in the water had dropped to normal – i.e., to the level observed before treatment – after one year. The corresponding period for biota was two to three years [21]. A two-year mesocosm study was conducted prior to the Björnöfjärden treatment in order to evaluate the impact of the method on brackish water ecosystems. The treatment increased the aluminium content in the water, but not in the organisms. No measurable toxic effects on mortality, primary production and respiration were detected [22]. However, it should be emphasised that further research on the effect of long-term exposures is necessary.
Another aspect to take into account is the fact that sediment treatment may disturb the sediments and result in the release of contaminants, although this has not been documented. That is why it is important to know before treatment whether such contamination is present, as this may affect the way in which the measure is implemented.
Finally, the production of the aluminium solution used in precipitation is energy intensive as the raw material has to be mined [24]. Compared to other measures, the carbon footprint of aluminium precipitation is high and at the same level as that for dredging (Table 8).
Possible combinations
In the event of mass occurrence of benthic fish species such as bream, it may be appropriate to combine the method with reduction fishing in order to reduce the bioturbation of sediment material [9, 25]. Such a combination could enhance the effectiveness of the measure against internal loading and reduce the risk of resuspension and transport of the flocculated material.
Documented experience
Lakes – The method has been used for 50 years in a number of lakes in Sweden and elsewhere. An evaluation shows that the measure has produced mixed results over time; no improvement was seen in some cases, while in other lakes the improvement continued for decades. The amount of aluminium added was found to have a significant impact on the outcome of the measure. The method has also been deemed to be cost-effective [3, 26, 27, 28].
Lake Långsjön (Stockholm), which is shallow and unstratified, was treated with polyaluminium chloride in 2006, which significantly reduced the phosphorus concentration in the water body [1, 29]. The dose of aluminium to Långsjön was based on the mobile phosphorus content of the sediment. In recent years, however, there has been deterioration in the form of reduced water transparency and an increase in total phosphorus and chlorophyll content, probably due to continued high loading from external sources. Near Långsjön is lake Flaten, which was treated back in 2000. There are no signs of deterioration here in terms of phosphorus levels in bottom or surface waters [1, 30]. The external load is low, which probably explains the difference in the lifetime of the measure compared to Långsjön. These two lakes are a good example of how external phosphorus inputs must be reduced sufficiently if internal loading measures are to have a long-term effect.
In 2017, the municipality of Växjö was given a ten-year licence to treat bottom sediments in the lakes Växjösjön and Södra Bergundasjön with aluminium. This treatment is the first of its kind to be reviewed for a licence by a Swedish court [31] (Judgment M2666-16). A licence was granted to distribute a total of 60 tonnes of aluminium (no more than 36 tonnes per treatment session) in Växjösjön. For Södra Bergundasjön, the licence allowed a total of 500 tonnes of aluminium to be distributed (no more than 300 tonnes per session).
The conditions for the licence were that:
- The precipitation element, polyaluminium chloride, must contain such low levels of unwanted trace elements that it is approved for drinking water production.
- Distribution of aluminium is permitted only when the pH of the lakes is within the 6.2–8.5 range. If the pH of the lakes goes outside the 6.5–8.0 range, the supervisory authority must be notified: they may then impose any additional conditions for enhanced monitoring that may be necessary.
- The alkalinity must be >0.2 meq/L.
- To protect bird life, aluminium treatment barges must not operate within specific protected areas or at specific times.
It is of interest to note that when Växjösjön was treated, the amount of aluminium and heavy metals in the water body decreased significantly due to the reduced amount of particles in the water.
Brackish water – Sediment injection of aluminium has also been carried out (in 2012 and 2013) in a brackish water environment in Björnöfjärden in the municipality of Värmdö [32, 33]. Björnöfjärden has a limited water exchange due to a narrow estuary towards the archipelago. A total of 36 tonnes of aluminium (50 g Al/m2) was injected into the top layer of sediment on bottoms less than 6 m deep, where oxygen was in short supply. Subsequent measurements showed that the concentration of total phosphorus in Björnöfjärden was reduced by about half compared to the level before the measure and compared to a reference bay nearby. After the measure, the water quality could be classified as high, with good status in respect of total phosphorus, chlorophyll and water transparency [34]. However, the bottom water was still low in oxygen for a couple of years after the treatment due to an oxygen debt following high plankton deposition in previous years. Oxygen consumption is expected to decrease after reduced deposition of organic matter [32]. The phosphorus concentration in the bottom water was reduced by 90%, indicating a positive intervention effect where the sediments bind phosphorus despite the low oxygen conditions at the bottom [33, 35].
The cost of this measure varies depending on how much phosphorus needs to be inactivated, the size of the lake and whether water treatment or sediment treatment is used [81]. The Swedish water authorities’ report on measures against eutrophication [28] used a standardised cost of SEK 48,000 per hectare. This can be compared with the cost of treating Växjösjön and Södra Bergundasjön in the municipality of Växjö, which is estimated at SEK 83,000 and SEK 50,000 per hectare respectively, or SEK 1,600 and SEK 750 per kg of phosphorus. The cost of measures for these lakes relates to sediment treatment. The cost of sediment treatment in Björnöfjärden totalled about SEK 9 million, or SEK 2,250 per kg of phosphorus, which largely consisted of development costs [21]. A study [26] of aluminium precipitation in the water column of four American lakes reported a mean cost of about SEK 245 (USD 27) per kg of fixed phosphorus. However, the cost range was wide (USD 14–95 per kg P).
Important matters to consider when designing the measure
Three things are important for correct design of an aluminium treatment:
- Calculate the amount of releasable/mobile phosphorus in the active sediment layer.
- Ensure that the pH of the aqueous phase does not fall below 6 during and after treatment.
- Maximise the amount of phosphorus bound to the mineral.
Sediment samples divided into multiple layers (see Appendix 4) need to be collected and analysed in order to calculate the amount of mobile phosphorus in the sediment, and to calculate the active sediment depth (the part of the sediment that releases phosphorus to the water column).
Geochemical modelling must be performed using water chemistry data from the lake and the anticipated aluminium dose. Free software is available (e.g., PHREEQC from USGS) that can be used for this. Alkalinity, pH, soluble Al forms, etc. can be modelled to simulate conditions during treatment, and to calculate the aluminium dose that can be added without risking harmful effects to the environment.
The binding ratio (Al:Al-P) in lakes treated previously has varied greatly over time, from 2:1 to over 20:1. The binding efficiency can be modelled using empirical models [8, 81]. If a particular aluminium dose is expected to have poor binding efficiency, it is better to divide the treatment into a number of treatments every few years.
2 Clay with added phosphorus-binding substances
Clay with added phosphorus-binding substances such as aluminium or lanthanum is added by dispersal in the water body. Full-scale experiments using a mixture of bentonite clay and lanthanum were conducted for the first time in 2001 [36]; and more experiments have been carried out since then in both lakes and laboratories.
Method description
A mixture of bentonite clay and phosphorus-binding substances such as aluminium or lanthanum, for example, is added to water and forms a sludge that binds phosphorus as it sinks to the bottom. Once at the bottom, the sludge settles on the sediment surface where phosphorus binding can continue. The bond between lanthanum and phosphate is strong, and in theory each lanthanum ion added should be able to bind one phosphate molecule, i.e. there is a 1:1 ratio of phosphate to lanthanum. As with aluminium treatment, phosphorus is also bound under anoxic conditions. It has been suggested that when using a clay material containing 5% lanthanum, for example, that a dosage of one tonne of clay material with the added lanthanum should be used to bind 11 kg of phosphate [37].
Location
The binding capacity of lanthanum-modified bentonite (LMB) material has been shown to be negatively correlated with alkalinity [38], i.e. it works best in soft water with low alkalinity. The binding capacity decreases at higher pH (pH >8) [38, 39]. This method is not suitable in lakes where extensive resuspension of the sediments occurs [40] such as shallow, unstratified lakes or lakes with extensive bioturbation.
There are studies indicating that the method achieves poorer results in waters containing high levels of humic substances [45]. This is thought to be due to lanthanum complexing with humic substances, which reduces the efficiency of LMB in binding phosphorus. The amount of lanthanum released from the clay increases as the concentration of dissolved organic carbon (DOC) approaches 8 mg/L. At this level of DOC, the amount of phosphorus bound to the clay is reduced by between 18 and 34% [82].
Timing
To remove the maximum amount of phosphorus from the water body, one manufacturer of LMB recommends that treatment should be carried out when the phosphorus is in a form that binds to LMB, i.e. as phosphate or as filterable reactive phosphorus. For northern Europe, this means the period from early autumn to early spring [41]. However, LMB continues to bind phosphate as long as there are active binding surfaces. It should therefore be possible to determine the timing of the measure on the basis of similar criteria to those used to calculate the appropriate timing of aluminium precipitation.
Disadvantages and risks
Lanthanum is toxic in higher concentrations. However, lanthanum does not occur as La3+ ions at neutral or alkaline conditions, i.e., the prevailing pH ranges in eutrophic lakes. A summary of toxicity measurements from 16 experiments in lakes shows that lanthanum levels are low in water with an alkalinity of >0.8 mEq/L [42]. Impacts on Daphnia were observed in lakes with very low alkalinity. However, it was not possible to establish whether this was due to the lanthanum levels, as it could also have been due to the dissolved particles formed when LMB was added [43].
The toxicity of one modified clay, PhoslockTM, has been tested on a number of different species. One example is a Canadian study where the LC 50 (median concentration causing 50% mortality) for Daphnia magna (48h) and LC 50 (96h) for rainbow trout were >4.9 g/L and >13.6 g/L respectively. This was more than 100 times the dose applied in the lake in question (0.02–0.05 g/L) [44].
This method results in the accumulation of bentonite on the bottom and in sediments, which could have an adverse impact on the ecology of the sediments. However, there is no detailed data on this in the scientific literature. Studies have shown a relationship between LMB and ammonium nitrogen emissions [46, 47].
Producing lanthanum is energy-intensive as the raw material has to be mined, which is a disadvantage from a sustainability perspective. However, there are currently no detailed life cycle assessments for internal loading measures, which makes it difficult to compare the economic and environmental sustainability of various measures.
Possible combinations
This measure should be preceded by reduction fishing if benthic fish can be expected to cause extensive bioturbation.
Documented experience
There is experience with the method in several countries, but no meta-analysis of the results has been carried out as yet. In Scotland, large-scale treatment [48] was carried out using the manufacturers’ recommended dosage (24 tonnes of PhoslockTM to bind about 270 kg of phosphorus). No change in mobile phosphorus levels could be observed after 28 days. The article concludes that PhoslockTM was underdosed in relation to the amount of mobile phosphorus. Another study [38] shows that the dosage recommended by the manufacturers may be too low to achieve the anticipated effect.
Important matters to consider when designing the measure
Modified clays are affected by dissolved organic carbon in the water, and so this must be taken into account. There are empirical models that calculate the deterioration of the binding efficiency [43]. It is possible that divided doses would improve the binding efficiency slightly, as with aluminium treatment, but no data are available to confirm this at present.
3 Bottom water withdrawal
Bottom water withdrawal or hypolimnetic withdrawal involves withdrawal of deep water containing high concentrations of phosphorus. This method is best suited to stratified lakes and is an inexpensive and less complicated alternative for addressing internal loading. However, this assumes that extensive temperature changes in the bottom water are avoided, and that the withdrawn water is dealt with in a way that does not adversely affect downstream water bodies. This method has been used in a number of lakes, mainly in Central Europe, since the mid–20th century [49].
Method description
Removal of hypolimnetic water means draining bottom water containing high phosphate concentrations that would otherwise remain near the sediments under stratified conditions. Hypolimnetic withdrawal also reduces the turnover time of the bottom water if this is done during a stagnation period, which means that oxygen conditions at the bottom can be improved. Internal loading is therefore countered from two directions: firstly, by the direct removal of phosphorus; and secondly, by improving oxygen conditions so that phosphorus can stay bound in the sediment.
The hypolimnetic water can be carried downstream by means of the siphon effect, or by active pumping. Alternatively, the bottom water is pumped up and may, for example, be used for irrigation purposes [50]. However, bottom water should be discharged in such a way so as to maintain the water level and stratification [51] thus avoiding effects on fish and other animals that are reliant on stratified, cooler water in summer. The water level can be controlled by withdrawing only a water volume equivalent to the annual surface water runoff, or by partially compensating for the withdrawal with new water of better quality.
Bottom water must be withdrawn at a sufficiently low flow rate so as not to cause mixing and thus affect stratification. For optimisation, the pipe withdrawing the bottom water should be placed at a depth with high phosphorus concentrations, as deep as possible without disturbing the sediments when withdrawing the water. The effectiveness of this measure increases according to the amount of bottom water removed, provided that the stratification and water level are maintained [51]. The end of the pipe may terminate at a lower level than the inlet in cases where the siphon effect is to be employed.
Location
Withdrawal and pumping of hypolimnetic water can be used as a restoration method in stratified lakes where large amounts of phosphorus have accumulated in the hypolimnion [49]. Even well-defined bays with stable stratification can be pumped to remove bottom water.
This method has been described as useful in deep, stratified and relatively small lakes [52], but unsuitable for unstratified lakes that do not develop a stable deep-water layer with high phosphorus concentrations [49, 53].
When pumping, it is important to investigate local conditions for disposal of the water. The impact on the recipient must be analysed if the water is diverted. Therefore, it may be necessary to treat the withdrawn water by means of filtration, for example, before returning it to the recipient.
Timing
This measure should be implemented during the seasons when the concentration of phosphorus in the bottom water is at its highest (summer, early autumn and late winter), but before the water mixes and the stratification is weakened by for example strong winds in autumn [51, 52]. Withdrawal should begin in summer when the water is stratified, but before the hypolimnetic water has become anoxic and hydrogen sulphide and Fe2+ have accumulated in the bottom water [51].
Disadvantages and risks
If water is channelled downstream, the downstream recipient will be affected. Effects may include eutrophication, changes in temperature, increased consumption of oxygen and the development of unpleasant odours [49]. There is also a risk that toxic substances may have accumulated in the bottom water. Therefore, the hypolimnetic water must be managed in such a way that there is no risk of deterioration of water quality downstream of the measure. Irrigation is a possible solution, water quality permitting. Otherwise, it may be necessary to filter or otherwise treat the withdrawn water.
If the water is disposed of on land it may also be necessary to investigate the potential negative effects of the nutrient-rich and – in some cases – saltier bottom water. An impact assessment of this kind should also include analysis of the risk of groundwater contamination.
One significant risk with hypolimnetic withdrawal is that the temperature in the hypolimnion may increase and stratification weakened. In this case, oxygen deficiency in the bottom water may occur or become more extensive while mixing in the lake increases. Increased mixing during the productive part of the year may exacerbate eutrophication as nutrients can then be transported upwards to the epilimnion and stimulate algal blooms.
This method needs to be repeated over a number of years in order to have a lasting impact, but it can be difficult to calculate the number of years in which the measure needs to be repeated in order to reduce diffusible phosphorus to a level where the measure can be discontinued.
Possible combinations
Experiments are in progress in Lake Kymijärvi in Finland, where the water pumped up is treated with different filter materials and then returned to the lake via a wetland.
Withdrawn hypolimnetic water can be used for irrigation in some cases (see below). It is also possible to combine the measure with filtration of the bottom water in order to remove nutrients before it is returned.
Documented experience
A pilot study was conducted at Bornsjön lake in 2004 where the bottom water was pumped away to reduce the internal phosphorus load [54]. Pumping took place during the stratified period, June to November, from the north-western basin of Bornsjön at the outlet to Lake Mälaren. No clear change in phosphorus concentrations was observed in the pilot study. However, more stable consumption of oxygen – which had previously been accelerating – was noted. It was suggested that a more efficient pump would give better results. The period during which the measure was implemented was brief, compared to trials lasting several years. An evaluation was performed in 2012 in order to determine the most appropriate method for reducing internal loading throughout Lake Bornsjön [55]. Bottom water pumping was suggested as a better measure than either hypolimnetic aeration (see the section on oxygenation below) or aluminium precipitation. The arguments in favour of pumping bottom water included the strong stratification of Lake Bornsjön, which reduces the risk of mixing, plus the fact that the existing water treatment plant can be used to treat the bottom water.
Lakes in southern Europe and elsewhere have been treated successfully in terms of reducing total phosphorus concentration. The study of these lakes showed that the concentration of total phosphorus in the epilimnion was significantly reduced after 2–3 years of withdrawal [51]. This measure has also been shown to reduce the concentration of phosphorus in the bottom water of stratified lakes [49].
This method is currently being tested in Lake Dynestadsjön, at the far end of Gamlebyviken Bay, which suffers from extensive eutrophication and has very limited mixing [50]. The high nutrient concentrations also affect the adjacent bay. That is why nutrient-rich bottom waters from Dynestadsjön have been pumped up and used to fertilise nearby farmland. A laboratory study gave good results in respect of growth of test crops irrigated using bottom water [56]. 50 ha of arable land and 10 ha of pastureland were then irrigated with a total of 59,760 m3 of water from Dynestadsjön between April and August 2018. The nutrient content of 20 g of nitrogen and 2 g of phosphorus per m3 of removed lake water meant that approximately 1,250 kg of nitrogen and 125 kg of phosphorus were likely absorbed during the summer of 2018. The nutrients correspond to about 15% of what the crop needs, and all the water, nitrogen and phosphorus pumped up were also absorbed by the crop [57].
For many years, nutrient-rich and oxygen-poor bottom water from Brunnsviken Bay in Stockholm has been pumped out to Lilla Värtan in order to reduce the extent of oxygen-free bottom water, thereby reducing the diffusion of phosphorus from the bottoms. This measure has reduced phosphorus levels in the bottom water of Brunnsviken Bay and also in the surface water, but oxygen levels remained low in 2017 and hydrogen sulphide was formed in the deep areas. The measure also worsens eutrophication in Lilla Värtan Bay [58].
Important matters to consider when designing the measure
It is very important to avoid breaking the stratification when withdrawing water. If this happens, the measure will be ineffective and there is a risk that the water quality may even deteriorate due to increased transport of phosphorus from bottom water to surface water.
4 Dredging
Dredging involves the removal of sediment from the water body. By removing the upper most nutrient-rich layer of sediment, internal loading of phosphorus may be reduced. The measure is described only briefly in this guide, as detailed criteria for dredging are addressed in the Swedish Agency for Marine and Water Management’s guide on dredging and management of dredged material.[59].
Method description
Water-rich sediment is removed by means of suction dredging, or by conventional dredging. Dredging removes oxygen-consuming and phosphorus-rich material from the top layers of the sediment, and internal phosphorus loading is thus reduced.
Normally the sediment is dewatered, and the discharged water treated to remove nutrients and any pollutants before it can be returned to the lake. The dredged material can be used as a soil improver if it is free of pollutants and heavy metals. However, if the dredged material is categorised as hazardous waste, it must be handled and disposed of safely, which can be costly.
For successful restoration, dredging should be carried out relatively quickly and target a majority of the bottom surface covered by sediments with a high amount of mobile phosphorus [60]. This avoids distributing material from undredged areas to the dredged areas, which reduces the effectiveness of the method. For dredging to remove the sediment layers that are the source of internal loading, it is important to know to what depth in the sediment excess mobile phosphorus is present [60].
Location
This method can be used in both shallow and deep lakes [61] where internal phosphorus loading is significant and the dominant source of the eutrophication problem. The method is best suited for water bodies where the bottom contains an identifiable upper sediment layer with significantly higher amounts of mobile phosphorus.
Timing
Dredging should be carried out outside the period when fish migrate or spawn in the area in question [62].
Disadvantages and risks
Dredging is an expensive, but also effective method when done properly [14] [14]. The method also means that dredged material, its nutrient content and any pollutants need to be dealt with on land, which adds to the costs. Moreover, dredging operations require large areas for sediment dewatering ponds. In the runoff process in these ponds, the phosphate in the discharge water can be precipitated with aluminium and the water can be pumped back to the lake [63]. Dredging causes the sediment to be suspended in the water, which – at least temporarily – increases nutrient diffusion and can impair water transparency, bottom fauna and underwater vegetation [63]. Dredging also risks exposing deeper layers of mobile phosphorus. That is why it is important to analyse the depth of mobile phosphorus in the sediment before embarking upon the measure. This measure may also increase diffusion of metals and organic pollutants into the water column that would otherwise be permanently buried (see [64], as an example).
The above description of disadvantages and risks should merely be regarded as a supplement to the Swedish Agency for Marine and Water Management’s guide on dredging and management of dredged material [59]. Therefore, please refer to this for more detailed information on dredging.
Possible combinations
Dredging can be combined with biomanipulation. This combination may be appropriate if it is necessary to restore aquatic plants after dredging, for example.
Documented experience
Dredging has been carried out in several lakes and large ponds, with varying results. Restoration of Lake Finjasjön in Hässleholm failed, partly because the sediment depth for mobile phosphorus was poorly defined and resuspension in the large, shallow lake moved nutrient-rich sediment to the areas that had already been dredged [60].
Lake Trummen outside Växjö was dredged in the 1970s in order to address eutrophication problems. This restoration was considered successful, as both phosphorus and algae levels were reduced by 50–70% [14, 63]. However, the macrophyte vegetation in Trummen was adversely affected and has still not recovered. Macrophytes are important for reducing the risk of algal blooms, and thus restoration efforts are ongoing [65].
Important matters to consider when designing the measure
There are a number of steps involved in designing a dredging project. These are just a few important things to consider:
When assessing the depth to which dredging is required, it is important to work on the basis of the profile of the mass of phosphorus in the sediment and not the phosphorus concentration. The units mass (mg/cm3) and concentration (mg/g dry weight) are different measures, and the mass of phosphorus, not the concentration, is what drives internal loading [83]. The concentration of phosphorus is usually highest at the sediment surface, but the mass of phosphorus may be highest in slightly deeper sediment layers. This is because the top few centimetres of sediment usually have a very high water content (about 95%), which means that there are not many sediment particles and therefore less phosphorus mass even if the concentration is high. The concentration indicates how much phosphorus is bound to the sediment particles, while the mass indicates how much phosphorus is present in a given volume of sediment. For example, if the 0–1 cm layer has a water content of 95% and 4 mg/g of phosphorus in the sediment while the 1–2 cm layer has 90% water and 2 mg/g of phosphorus, the two layers have the same phosphorus mass in mg/cm3.
Sediments have a high water content. In eutrophic lakes with high levels of organic matter in the top sediment layer, this layer often has a water content of > 90% in the zones in which sediment accumulates. By way of comparison, soured milk (for example) has a water content of about 88%. It is possible to draw a track in a bowl of soured milk with a spoon and watch the track filling up straight away. Even the outer layers of sediment can move quite easily and quickly, so dredging needs to be designed to reduce the risk of filling the tracks with sediment from the sides.
There may be contaminants in the sediment to be dredged, but they may also be present below the dredging depth, i.e., in the new sediment surface. That is why it is important to also analyse the sediment that will be exposed to the water after dredging. Some heavy metals form stable substances under low oxygen conditions. These may dissolve when exposed to oxygen after dredging, and the metals can be transported to the water body. There may also be organic contaminants in deeper sediment layers that need to be taken into account.
It is important not to remove all the seeds from macrophytes in the lake as these are needed to allow macrophytes to re-establish themselves after dredging has taken place. Removal of macrophytes and seeds is what caused the loss of macrophytes in Lake Trummen in Växjö. Macrophytes are an important part of a lake’s ecosystem, and they also stabilise the sediments. Seeds are less likely to be removed if only the first few cm of sediment is dredged, but there is a risk of a great reduction in the amount of macrophytes if the dredging depth is >10–20 cm. Low-flow dredging is less likely to inadvertently remove macrophyte seeds.
There is also a risk that bottom fauna will be removed and killed, so it is important to take this into account and retain areas where bottom fauna can survive and re-establish. Low-flow dredging is less harmful to benthic organisms than conventional dredging.
5 Reduction fishing (biomanipulation)
Reduction fishing is carried out primarily to increase the grazing pressure on phytoplankton by removing fish that eat zooplankton. This is a common measure applied in eutrophic lakes [10]. Moreover, benthic fish foraging in sediments are of particular importance for internal phosphorus loading. This is because foraging mixes the top layer of sediment, allowing more mobile phosphorus to be released from the sediment into the water column [10, 66]. This method targets species known as white fish, i.e., cyprinids such as roach and bream.
Method description
Many attempts have been made in recent decades to restore eutrophic lakes by means of reduction fishing. A systematic review of this restoration method was published in 2015 [10]. The method is implemented in lakes where the composition of the fish population is dominated by white fish. Extensive and targeted fishing is carried out with a view to decimating the abundance of zooplankton-eating fish and fish foraging in sediments. Benthic fish that forage in sediments are of particular significance for internal phosphorus loading as foraging mixes the top layer of sediment, allowing more mobile phosphorus from the sediment to be released to the water column [9, 10, 66].
Cyprinids such as roach or bream are usually reduced in number, and there is a positive correlation between the extent of removal of these fish and the effectiveness of the measure. At least 80% of the white fish should be removed over a period of 1 to 3 years. This can correspond to extraction of up to 200 kg fish per ha in nutrient-rich lakes, but this figure varies widely. Targeted fishing for bottom-feeding fish such as bream should be given particularly high priority. Experiences from lake Ringsjön have been that the aim should be that after reduction fishing fewer than 20 bream are caught per net and no more than 15–20% perch (>10 cm) [67].
When done properly, reduction fishing can result in significant positive results on water quality when depleting fish stocks, and for a number of years afterwards [10, 68]. When implementing this measure, efforts should be made to release any by-catch of predatory fish as these help to reduce the dominance of cyprinids. The possibility of fish migration between lakes in the catchment area should also be taken into consideration when planning for reduction fishing. The effectiveness of the measure may be adversely affected if there is mass migration of cyprinids between adjacent lakes [69], for example. There are different fishing methods, and the method that should be selected is dependent on the fish targeted and the local conditions. Trawling and seine netting were the main methods used in Ringsjön (municipalities of Höör, Hörby and Eslöv), for example, but pound nets and fyke nets were also used [67].
The ability of macrophytes to re-establish themselves over large areas of the lake bed is an important factor in the lasting effect of reduction fishing [84, 85].
Location
This method is suitable for waters dominated by zooplankton-eating (zooplanktivorous) and benthic fish. According to a systematic evaluation, reduction fishing is most successful in small lakes with a short turnover time and high phosphorus levels before the intervention [10]. Potential explanations for this, according to the study, included the fact that it is easier to remove large parts of the fish population in a smaller lake. It is more difficult to explain why turnover time correlates with the effect of the measure. However, previous studies have suggested that for the most part, shallow lakes without stratification are best suited for reduction fishing as the potential for recolonisation of macrophytes is greater in such lakes (see, for example, [9], [84]. In [10], data on stratification are missing for about half of the lakes in the study, which could at least partly explain the difference in the conclusions. The effect of the measure thus varies, depending on both the extent of the implementation and the other characteristics of the lake; but long-term effects have been achieved in both deep, stratified lakes and shallow, well-mixed lakes.
Timing
Reduction fishing can be initiated in the spring, for instance, starting just after the thaw, corresponding to the time before and during the fish spawning period, but it can also be started at the end of the year if the conditions are right. Reduction fishing using seine nets has also been carried out in winter when the water is frozen over. In summer, it may be appropriate to suspend reduction fishing if there are high water temperatures of about 25 °C or above so as to avoid causing unnecessary stress to predatory fish that are caught as by-catch and need to be released back into the water [70].
Disadvantages and risks
This measure may need to be repeated at regular intervals in order to maintain the improved water quality, which may otherwise be affected by factors such as new recruitment of white fish, continued diffusion of accumulated phosphorus from sediments, insufficient measures to reduce external nutrient loading or under-representation of macrophytes [10, 66, 71]. Moreover, the lack of long-term measurements makes it difficult to draw conclusions about the effects of biomanipulation beyond three years after the start of the measure. However, there are cases in which the measure has been effective for a decade or more [10].
It is important to use selective fishing gear that allows only the fish targeted by the measure to be removed, otherwise there is a risk of the measure being counterproductive.
Possible combinations
When carrying out reduction fishing, predatory fish are sometimes set out so as to further reduce the dominance of cyprinids. However, only setting out predatory fish has not been shown to result in significant improvements in either water transparency or chlorophyll content [10].
Reduction fishing may be an appropriate method to combine with methods for the permanent fixation of phosphorus, such as aluminium precipitation [25, 69]. The planned low flow dredging to be carried out as part of the restoration of Lake Barnarpasjön (municipality of Jönköping) will be supplemented by reduction fishing [72].
Documented experience
Reduction fishing has been carried out in many lakes for a long time. Some recent examples are given below.
In Ringsjön, reduction fishing was carried out in 1988–1992 and has subsequently been carried out continuously since 2005, with a total catch of just over 1,100 tonnes of white fish after 2005. This has resulted in noticeable improvements in water quality. The nutrient concentrations are lower than before the measure began, and significant improvements in both water transparency and macrophyte distribution have been achieved. It has also been noted that algal blooms have become less intense and the amount of zooplankton has increased. That said, it is recognised that there is a need for continued maintenance fishing to enable the continued expansion of underwater plants.
The municipality of Växjö has conducted reduction fishing in Trummen, Växjösjön and Södra Bergundasjön, the three lakes close to the city, between 2016 and 2018. About 200 tonnes of fish were removed from the lakes in total, and the initiative has improved water quality in all the lakes. The degree of improvement differs between the lakes, which is explained by the different levels of internal loading. Reduction fishing is considered sufficient for Trummen, but in Växjösjön and Södra Bergundasjön the measure has been supplemented with aluminium precipitation. The total cost of reduction fishing amounted to about SEK 3.5 million. Most of the catch was delivered to the biogas plant for the municipality of Växjö, and the market value of the biogas produced was estimated to be around SEK 0.7 million. Neither this value nor the climate benefits of biogas production have been included in the total cost of the measure [69].
In Lake Vallentunasjön, reduction fishing has been ongoing since 2010 but has not resulted in clear improvements in water quality: see [73], for example. One reason for this may be the strong internal loading of the lake, which results in a fourfold increase in the total phosphorus content of the lake’s water body in spring and early summer. A total of 195 tonnes of white fish have been harvested. This catch represents a large percentage of the phosphorus leaving the lake via the outlet, assuming that the phosphorus content of the white fish was 0.7–1% of wet weight. The additional phosphorus export from the lake in the form of fish biomass was thought to be capable of accelerating the evolution of the lake towards a less eutrophic state [73].
Many lakes in Denmark and the Netherlands have undergone reduction fishing, and a summary of more than 70 projects in mostly shallow and eutrophic lakes was published in 2007 [71].
Important matters to consider when designing the measure
There is a risk that the measure may remove too much white fish, which – combined with a reduction in nutrient levels – may make it difficult for predatory fish to find sufficient food. This measure reduces the amount of phytoplankton, which can have a knock-on effect throughout the food chain. Unfortunately, there is not much information on how a fish community needs to be structured following measures to reduce stress on predatory fish. All we know is how such a community is structured under natural conditions, when there is a balance. One possibility is to estimate the lake’s natural fish biomass bearing capacity (i.e. if the lake were unaffected by eutrophication) and use this to estimate the amount of white fish and predatory fish that can be removed by means of reduction fishing.
6 Mixing
This method involves mixing the water column to oxygenate the water throughout the lake depth, thereby increasing the oxygen concentration in the bottom water. The aim of this is to increase the retention of phosphorus in the sediments. Mixing may, however, cause increased primary production of phytoplankton and there are no successful examples of its application that show that the method has been successful. The method and the underlying principle have been questioned in recent decades.
Method description
Mixing is performed by means of a pump that pushes air or oxygen-rich water to a depth below the thermocline or near the bottom. Pumped water or air rises through the water body and induces a vertical flux while causing turbulence and mixing with the surrounding water. Besides pumps, propellers can be used to create vertical movement and turbulence in the water, thereby mixing the water column. More force is required for mixing in stratified waters. This measure may also aim to prevent stratification in unstratified waters.
The method is used to mix the entire water body, primarily in winter. The aim is to prevent oxygen deficiency at the sediment surface in order to allow phosphorus to be naturally bound to the sediments. However, experience has shown that the effect of mixing in lakes can be to increase primary production instead [14, 53].
Location
This method has potential in waters where preconditions exist for phosphorus fixation in the sediment (e.g. sediments containing dissolved reduced iron) as oxygen conditions at the bottom will improve. The measure has not given satisfactory results in heavily eutrophic lakes [74].
Timing
The method has been used in different ways in different seasons. The entire water body can be mixed with relatively little effort in autumn or winter, when stratification is weaker. In lakes, for example, the method has been implemented by using more pumps in the summer to break the stratification [75], and by reducing the airflow to maintain the stratification and thus prevent nutrient diffusion to shallower water layers during the summer [74]. None of these experiments had any effect on internal loading.
Disadvantages and risks
There have been cases where this method has been used in lakes without improving internal loading, and instead resulting in an increased flux of phosphorus from the bottom [74, 75]. Mixing can increase the temperature in the hypolimnion and also cause resuspension at the bottom, which increases the chances of phosphorus and other pollutants being released into the water column. Moreover, mixing can be periodically enhanced by strong winds in shallower water layers. Resuspension is caused in particular in shallow areas that are more mixed by wind.
While mixing certainly may increase the binding of phosphorus to oxidised iron, it also risks causing more phosphorus to be released from sediments. This is caused by increased mineralisation of organic matter due to increased oxygen supply, thereby increasing the availability of phosphorus for primary production. If the temperature increases as well, the rate of mineralisation also increases [60]. That is why this method has been associated with many potential risks, such as increased total phosphorus and algal blooms, when used in lakes [14].
Mixing cannot be expected to lead to permanent positive effects but has to be carried out continuously, and the potential of the measure is disputed [74, 75]. Moreover, large amounts of energy are required to pump large amounts of water or air vertically for a long time.
Possible combinations
Mixing may counteract the effectiveness of other methods, and so combining the measure with other methods in lakes is not recommended [14].
Documented experience
An evaluation of mixing in Lake Sempach and Lake Baldegg over a 10-year period has shown that the phosphorus flux from the bottom did not decline even though oxygen conditions at the bottom improved [74]. Similar observations have recently been made in Finland. Mixing using a pump in Lake Tuusulanjärvi has been carried out since 1998, but with no effect on the internal phosphorus load. On the contrary, mixing resulted in accelerated phosphorus flux from the sediments, despite improved oxygen conditions [75].
7 Oxygenation
The method that involves adding oxygen to deoxygenated bottom water has been used in lakes since the mid-20th century. The purpose of the measure is to oxygenate the bottom water so that the bottom is provided with access to oxygen and can thus bind phosphorus at the sediment surface. This measure is implemented by allowing bottom water to come into contact with oxygen or air. The measure can be viewed as easing the symptoms rather than remedying the causes of internal loading.
Method description
Oxygenation is used in lakes where phosphorus diffusion from the bottom is controlled by the release of iron-bound phosphorus as affected by the redox conditions at the sediment surface [76]. The method involves bringing the water in the hypolimnion into contact with pure oxygen (oxygenation) or air (hypolimnetic aeration) and then dissolving the oxygen.
Oxygenation of the bottom water is achieved by adding pure oxygen to the hypolimnion. The method of using pure oxygen is common as oxygen is more soluble than air, and so the method generates a high uptake of oxygen in the bottom water. Adding oxygen instead of air has several advantages, such as less turbulence because the high level of solubility means that only a small flow of oxygen is needed.
Oxygen can be supplied to the bottom water via a pump system that pumps oxygen from a reservoir down through a pipe and out into the hypolimnion. There, the oxygen rises and mixes with the bottom water until it reaches a level of equal density, where it then diffuses horizontally. To maintain stratification, the oxygenated bottom water must not rise above the thermocline. Another way to add oxygen is to submerge a contact chamber in the water. This contact chamber has access to oxygen, which is bubbled through the chamber, the bottom water being pumped through the chamber and thereby coming into contact with oxygen. Oxygen can also be added by pumping up bottom water on land, injecting oxygen and then returning the water to the bottom. This method has been described as beneficial compared to hypolimnetic aeration [77].
Hypolimnetic aeration is a method based on similar principles to oxygenation. Aeration is achieved by lifting bottom water to the surface using a pump or compressed air so that it comes into contact with oxygen in the air. The water is then transported back down to the bottom. Compressed air can be used to push bottom water up through a tube. The bottom water and air are then mixed in the tube in order to oxygenate the water before it is returned to the hypolimnion. The bottom water is either lifted all the way to the surface, or just partially. Hypolimnetic aeration can also be performed by bubbling compressed air into the hypolimnion.
If this method is designed properly, it allows a cool temperature to be maintained in the bottom water, and hence persistent temperature stratification. Maintaining this stratification is viewed as beneficial so as to prevent phosphorus from circulating from the bottom water to surface layers [76].
Location
In stratified lakes with sufficient water volume in the hypolimnion, oxygenation can to some extent reduce phosphorus in the hypolimnion [76]. The method should be avoided in shallow lakes where stratification is weak [52]. It is beyond the scope of this guide to indicate the specific water volume or depth at which the measure is appropriate, as this has to be examined and calculated in each individual case.
It is appropriate to conduct a survey of the sediment in question in order to analyse how much phosphorus binding material is available, and in what form. Oxygenation should not be carried out in waters where there is a risk of mobilising pollutants as a result of the measure.
Timing
Aeration should be conducted when a lake is stratified. Hypolimnetic aeration has been tested during winter and summer in Lake Tuusulanjärvi in Finland, but without improving the internal load on either occasion [75].
Disadvantages and risks
High circulation in the aeration system may be needed to successfully oxygenate the bottom water with hypolimnetic aeration. This may lead to turbulence, which can cause resuspension at the bottom. The turbulence may also cause mixing of the water body and destroy the stratification. That is why it is important that the oxygenation or aeration system is designed correctly. If not, the measure may in contrast increase phosphorus dispersal from the hypolimnion to the surface water [76].
Hypolimnetic aeration can be described as a method that reduces the symptoms of internal loading, rather than a method that involves the actual removal of phosphorus, as is the case with bottom water withdrawal [78]. A similar conclusion has been drawn from 10 years of restoration in two Swiss lakes, where compressed air was added to the hypolimnion in winter and oxygen was added in summer. This measure failed to affect the ability of the seabed to permanently fix phosphorus, and was therefore deemed to have no long-term effect [74].
It is important to examine whether oxygenation can cause mobilisation of pollutants, if there is reason to suspect the presence of such substances in the sediments.
Possible combinations
In Denmark, the method has been combined with reduction fishing [79].
Documented experience
Hypolimnetic aeration is a common way of restoring lakes in Finland and Denmark. Approximately 100 Finnish lakes are being treated using the method. The longest period of aeration in Lake Tuusulanjärvi has been ongoing since the early 1970s, and the measure is regarded by the general public as almost indispensable [80]. However, an evaluation of the measure has shown that internal loading in Tuusulanjärvi has not decreased, despite 40 years of treatment using different aeration methods [75]. In Denmark, hypolimnetic aeration has been used in four or five lakes, often in combination with reduction fishing and introduction of predatory fish [79].
In Lake Bornsjön, a reserve water source in Stockholm, hypolimnetic aeration has been applied in the eastern basin every summer since 1987. This aeration was stopped in 2004 and a dramatic increase in the amount of phosphorus in the bottom water was observed due to the deterioration of oxygen conditions at the sediment surface. This change indicated that phosphorus accumulated in the sediments can diffuse into the water as soon as aeration is stopped. That is why hypolimnetic aeration was not deemed to be a measure sustainable in the long term for Bornsjön [55].
Important matters to consider when designing the measure
For oxygenation to have the desired effect, it is important to ensure that there is sufficient binding capacity in the sediment to be able to bind the entire surplus of mobile phosphorus that is present there. If this is not the case, it is necessary to add aluminium- or iron-based binding materials before commencing oxygenation.
Previous studies have shown that sufficient iron must be present in order to bind diffusible phosphorus and prevent internal loading from the sediment under oxygen-rich (oxic) conditions (e.g. [86]). It is roughly estimated that the ratio between total iron and total phosphorus in the sediments needs to be higher than 15 for oxygenation to have the desired effect.
It may be necessary to carry out a number of laboratory-scale experiments on sediment samples to ensure that the measure will work. One way of testing the suitability of the measure is to add phosphorus to sediment samples (the top 4–10 cm of sediment) in increased amounts. This method allows the equilibrium phosphorus concentration (EPC0) to be calculated under oxygen-rich conditions, i.e. the concentration of phosphorus in lake water where sediments do not bind or release phosphorus. Oxygenation would probably be successful if the reference (target) phosphorus concentration to be reached is higher than this concentration. However, if the reference concentration is lower than the equilibrium concentration, there is a risk that phosphorus will continue to be released even after oxygenation.
It is also necessary to calculate oxygen consumption in order to calculate how much oxygen or air will be required to properly oxygenate the water. A laboratory experiment can be conducted here using sediment cores with water on top in order to measure how much the added oxygen is consumed, and how quickly. Oxygen consumption in the sediment is normally the largest part of total oxygen consumption, but some consumption also occurs in the lake water. Even when the measure is designed correctly, it is important to remember that several documented experiences show that the measure has not succeeded in permanently fixing phosphorus, and therefore it has no long-term effect after oxygenation stops.
8 Refererences
[1] | Schütz, J., Rydin, E. & Huser, B., “A newly developed injection method for aluminum treatment in eutrophic lakes: Effects on water quality and phosphorus binding efficiency”, Lake and Reservoir Management, 2017. |
[2] | Steinman, A., Rediske, R. & Reddy, R. K., “The reduction of internal phosphorus loading using alum in Spring Lake, Michigan”, Journal of Environmental Quality, vol. 33, pp. 2040–2048, 2004. |
[3] | Huser, B. J., Egemose, S., Harper, H., Hupfer, M., Jensen, H., Pilgrim, K. M., Reitzel, K., Rydin, E. & Futter, M., “Longevity and effectiveness of aluminum addition to reduce sediment”, Water Research, 97, pp. 122-132, 2016 b. |
[4] | Rydin, E. & Welch, E., “Dosing Alum to Wisconsin Lake Sediments Based on in vitro Formation of Aluminum Bound Phosphate”, Journal of Lake and Reservoir Management, vol. 15, no 4, pp. 324–331, 1999. |
[5] | Jensen, H., Reitzel, K. & Egemose, S., “Evaluation of aluminum treatment efficiency on water quality and internal phosphorus cycling in six Danish lakes”, Hydrobiologia, vol. 751, no 1, pp. 189–199, 2015. |
[6] | Rydin, E., Huser, B. & Welch, E., “Amount of phosphorus inactivated by Al treatments in Washington lakes”, Limnol. Oceanogr., vol. 45, no 1, pp. 226–230, 2000. |
[7] | Rydin, E., “Potentially mobile phosphorus in Lake Erken sediment”, Water Research, vol. 34, no 7, pp. 2037–2042, 2000. |
[8] | Huser, B. J., “Variability in phosphorus binding by aluminum in alum treated lakes explained by lake morphology and aluminum dose”, Water Research, vol. 46, pp. 4697–4704, 2012. |
[9] | Huser, B. J., Bajer, P. G., Chizinski, C. J. & Sorensen, P. W., “Effects of common carp (Cyprinus carpio) on sediment mixing depth and mobile phosphorus mass in the active sediment layer of a shallow lake”, Hydrobiologia 763, pp. 23–33, 2016 a. |
[10] | Bernes, C., Carpenter, S. R., Gårdmark, A., Larsson, P., Persson, L., Skov, C., Speed, J. D. M. & Van Donk, E., “What is the influence of a reduction of planktivorous and bentivorous fish on water quality in temperate eutrophic lakes?”, Environmental Evidence 4:13, 2015. |
[11] | Huser, B. J. & Köhler, S., “Potential toxicity and chemical processes of aluminium addition for sediment phosphorus control in Östhammarsfjärden”, Swedish University of Agricultural Sciences, Sweden, 2012. |
[12] | Jensen, H. S., Egemose, S. & Andersen, F. Ø., “Restaurering af danske søer med aluminium”, Vand og Jord, pp. 47–50, 2016. |
[13] | Municipality of Växjö, Consultation document – aluminiumbehandling av bottensediment i sjöarna Växjösjön och Södra Bergundasjön, 2015. |
[14] | Cooke, G. D., Welch, E. B., Peterson, S. A. & Nichols, S. A., Restoration and management of Lakes and Reservoirs. 3rd edition, Boca Raton: CRC Press, 2005. |
[15] | Jönköping County Administrative Board, “Utvärdering av labilt aluminium – kalkningsverksamheten i Jönköpings län”, Jönköping County Administrative Board, Jönköping, 2009. |
[16] | Huser, B. J. & Rydin, E., “Phosphorus inactivation by aluminum in Lakes Gårdsjön and Härsvatten sediment during the industrial acidification period in Sweden”, Canadian Journal of Fisheries and Aquatic Sciences, vol. 62, no 8, pp. 1702–1709, 2005. |
[17] | Huser, B. J., “Phosphorus binding by aluminium in sediment: A tool for restoring water quality in the Baltic Sea and other brackish surface waters”, SLU Rapport 2014:05, 2013. |
[18] | Reitzel, K., Jensen, H. S. & Egemose, S., “pH dependent dissolution of sediment aluminum in six Danish lakes treated with aluminum”, Water Research, vol. 47, pp. 1409–1420, 2013. |
[19] | Wauer, G. & Teien, H-C., “Risk of acute toxicity for fish during aluminium application to hardwater lakes”, Science of the Total Environment, vol. 408, pp. 4020–4025, 2010. |
[20] | Poleo, A., Östbye, K., Öxnevad, S., Andersen, R., Heibo E. & Völlestad, L., “Toxicity of acid aluminum-rich water to seven freshwater fish species: a comparative laboratory study”, Environmental Pollution, vol. 96, no 2, pp. 129–139, 1997. |
[21] | Kumblad, L. & Rydin, E., “Levande kusts vitbok 1.0”, BalticSea2020, 2019. |
[22] | Kumblad, L., Rydin, E., Lilliesköld Sjöö, G. & Mörk, E., “Fosforfällning för en förbättrad skärgårdsmiljö – ett mesokosmförsök”, BalticSea2020, 2012. |
[23] | Svealand Coastal Water Association, “Svealandskusten 2020”, Svealand Coastal Water Association, Stockholm, 2020. |
[24] | Karlsson, M., Malmaeus, M. & Rydin, E., “Åtgärder mot internbelastning av fosfor i Hjälmaren – kostnad, nytta och konsekvenser”, IVL Swedish Environmental Research Institute – report number C 381, Stockholm, 2019. |
[25] | Jeppesen, E. et al, “Biomanipulation as a Restoration Tool to Combat Eutrophication: recent advances and future challenges”, Advances in Ecological Research, vol. 47, pp. 411–487, 2012. |
[26] | Huser, B. J., Futter, M., Lee, J. T. & Perniel, M., “In-lake measures for phosphorus control: The most feasible and cost-effective solution for long-term management of water quality in urban lakes”, Water Research, 97, pp. 142–152, 2016 c. |
[27] | Lürling, M., Mackay, E., Reitzel, K. & Spears, B. M., “Editorial – a critical perspective on geo-engineering for eutrophication management in lakes”, Water Research, 97, pp. 1–10, 2016. |
[28] | Gyllström, M., Larsson, M., Mentzer, J., Petersson, J. F., Cramér, M., Boholm, P & Witter, E., “Rapport 2016:19 Åtgärder mot övergödning för att nå god ekologisk status – underlag till vattenmyndigheternas åtgärdsprogram”, De fem vattenmyndigheterna i samverkan, Västerås, 2016. |
[29] | City of Stockholm, 5 October 2017. [Online]. Available at: http://miljobarometern.stockholm.se/vatten/sjoar/langsjon/langsjon-reduktion-av-intern-fosforbelastning/ |
[30] | Agstam-Norlin, O., Lannergård, E. E., Rydin, E., Futter, M. N. & Huser, B., “A 25-year retrospective analysis of factors influencing success of aluminum treatment for lake restoration”, Water Research, vol. 200, p. 117267, 2021. |
[31] | Swedish Environmental Protection Agency, 2017. [Online]. Available at: http://sverigesmiljomal.se/larande-exempel/restaurering-av-overgodda-sjoar-i-vaxjo/. |
[32] | Rydin, E., Kumblad, L., Wulff, F. & Larsson, P., “Remediation of a Eutrophic Bay in the Baltic Sea”, Environmental Science & Technology 51 (8), pp. 4559–4566, 2017. |
[33] | Rydin, E. & Kumblad, L., “Capturing past eutrophication in coastal sediments – towards water-quality goals,” Estuarine, Coastal and Shelf Science, vol. 221, pp. 184–188, 2019. |
[34] | Svealand Coastal Water Association, Resultat från karteringar 2001−2015 (p32−25). Svealandskusten 2016. ISBN: 978-91-980325-4-3, http://havet.nu/dokument/Svealandskusten2016.pdf. : (SKVVF), 2016. |
[35] | BalticSea2020, 19 April 2017. [Online]. Available at: http://balticsea2020.org/pressrum/601-projektet-levande-kust-har-bidragit-till-en-friskare-bjornofjard. |
[36] | Robb M., Greenop B., Goss Z., Douglas G. & Adeney J., “Application of Phoslock™, an innovative phosphorus binding clay, to two Western Australian waterways: preliminary findings”, Hydrobiologia, vol. 494, pp. 237–243, 2003. | ||
[37] | “About PhoslockTM”, Phoslock Europe GmbH, [Online]. Available at: http://www.phoslock.eu/en/phoslock/about-phoslock/ | ||
[38] | Reitzel K., Andersen, F. Ø., Egemose, S. & Jensen, H. S., “Phosphate adsorption by lanthanum modified bentonite clay in fresh and brackish water”, Water Research, vol. 47, pp. 2787–2796, 2013. | ||
[39] | Ross, G., Haghseresht, F. & Cloete, T. E., “The effect of pH and anoxia on the performance of PhoslockTM, a phosphorus binding clay”, Harmful Algae, vol. 7, p. 545–550, 2008. | ||
[40] | Schauser, I., Lewandowski, J. & Hupfer, M., “Decision support for the selection of an appropriate in-lake measure to influence the phosphorus retention in sediments”, Water Research, vol. 37, pp. 801–812, 2003. | ||
[41] | PhoslockTM, “Phoslock FAQ”, [Online]. Available at: http://www.phoslock.eu . | ||
[42] | Spears, B. M., Lürling, M., Yasseri, S., Castro-Castellon, A. T., Gibbs, M., Meis, S., McDonald, C., Mc Intosh, J., Sleep, D. Van Oosterhout, F., “Lake responses following lanthanum-modified bentonite clay (PhoslockTM) application: An analysis of water column lanthanum data from 16 case study lakes”, Water Research, vol. 57, pp. 5930–5942, 2013. | ||
[43] | Lürling, M. & Tolman, Y., “Effects of lanthanum and lanthanum-modified clay on growth, survival and reproduction of Daphnia magna”, Water Research, vol. 44, no 1, pp. 309–319, 2010. | ||
[44] | Watson-Leung, T., “PhoslockTM Toxicity Testing with Three Sediment Dwelling Organisms (Hyalella azteca, Hexagenia spp. and Chironomus dilutus) and Two Water Column Dwelling Organisms (Rainbow Trout and Daphnia magna)”, Ontario Ministry of the Environment, Etobicoke, 2009. | ||
[45] | Lürling, M., Waajen, G. & van Oosterhout, F., “Humic substances interfere with phosphate removal by lanthanum modified clay in controlling eutrophication”, Water Research, vol. 54, pp. 78–88, 2014. | ||
[46] | Zeller, M. A. & Alperin, M. J., “The efficacy of PhoslockTM in reducing internal phosphate loading varies with bottom water oxygenation”, Water Research X , vol. 11, p. 100095, 2021. | ||
[47] | van Oosterhout, F. & Lürling, M., “The effect of phosphorus binding clay (PhoslockTM) in mitigating cyanobacterial nuisance: a laboratory study on the effects on water quality variables and plankton”, Hydrobiologia, vol. 710, pp. 265–277, 2013. | ||
[48] | Meis, S., Spears, B. M., Maberly, S. C., O’Malley, M. B. & Perkins, R. G., “Sediment amendment with PhoslockTM in Clatto Reservoir (Dundee, UK): investigating changes in sediment elemental composition and phosphorus fractions”, Journal of Environmental Monitoring, vol. 27, pp. 123–131, 2011. | ||
[49] | Nürnberg, G. K., “Lake responses to long-term hypolimnetic withdrawal”, Lake and Reservoir Management, pp. 388–409, 2007. | ||
[50] | Municipality of Västervik, “Övergödningen som en resurs. Genomförande av åtgärder för minskat läckage av näringsämnen från Dynestadsjön till Gamlebyviken. Styrdokument 2013–2018”, 2013. | ||
[51] | Nürnberg, G. K., “Hypolimnetic withdrawal as a lake restoration technique”, J. Environ. Eng., 113, pp. 1006–1016, 1987. | ||
[52] | Bormans, M., Maršálek, B. & Jančula, D., “Controlling internal phosphorus loading in lakes by physical methods to reduce cyanobacterial blooms: a review”, Aquat Ecol, pp. 407–422, 2016. | ||
[53] | Nürnberg, G. K., “Hypolimnetic withdrawal as a lake restoration technique: determination of feasibility and continued benefits”, Hydrobiologia, vol. 847, no TBD, pp. 4487–4501, 2020. | ||
[54] | Lindvall, A. & Ulén, B., “Bortpumpning av sjöars bottenvatten för att minska internbelastningen av fosfor, erfarenheter från Bornsjön”, Vatten, 61, pp. 183–192, 2005. | ||
[55] | Nürnberg, G.K. & LaZerte, B., “Evaluation of hypolimnetic withdrawal as a possible treatment for the Bornsjön Reservoir internal phosphorous load”, Stockholm, 2012. | ||
[56] | Municipality of Västervik, Tema vatten i Västerviks kommun, Volume 1, Number 1, 3 February 2014. | ||
[57] | Municipality of Västervik, “Fördjupad uppföljning av projekt ’Övergödningen som en resurs’ – delrapportering av bidrag ur Havs- och vattenmiljöanslaget”, 2019. | ||
[58] | “Stockholms stad: Miljöbarometern”, 2017. [Online]. Available at: http://miljobarometern.stockholm.se/vatten/kustvatten/brunnsviken/brunnsviken-forbattrad-utpumpning-av-bottenvatten/ | ||
[59] | Swedish Agency for Marine and Water Management, “Muddring och hantering av muddermassor – vägledning och kunskapsunderlag för tillämpningen (Rapportnummer 2018:19)”, Swedish Agency for Marine and Water Management, Gothenburg, 2018. | ||
[60] | Swedish Environmental Protection Agency, “Kan Östersjön restaureras? Utvärdering av erfarenheter från sjöar. Rapport 5860”, 2008. | ||
[61] | Huser, B. J., Löfgren, S. & Markensten, H., “Internbelastning av fosfor i svenska sjöar och kustområden – en kunskapsöversikt och förslag till åtgärder för vattenförvaltningen”, Report, Swedish University of Agricultural Sciences, Department of Aquatic Sciences and Assessment, no 2016:6, 2016 d. | ||
[62] | Swedish Environmental Protection Agency, “Muddring och hantering av muddermassor”, Implementation and Enforcement Department, Swedish Environmental Protection Agency, Stockholm, 2010. | ||
[63] | Svensson, M. & Lindahl, J., “Åtgärdsprogram för Ringsjön”, MS Naturfakta, 2002. | ||
[64] | Yu, J., Chen, Q., Zhang, J., Zhong, J., Fan, C., Hu, L., Shi, W., Yu, W. & Zhang, Y., “In situ simulation of thin-layer dredging effects on sediment metal release across the sediment-water interface”, Science of The Total Environment, 658:501-509, 2019. | ||
[65] | Municipality of Växjö, “Vattenväxter Trummen – fältförsök 2014”, 2014. | ||
[66] | Søndergaard, M., Jensen, J. P. & Jeppesen, E., “Role of sediment and internal loading of phosphorous in shallow lakes”, Hydrobiologica, 509: 135–145, 2003. | ||
[67] | Nyström, P., Stenberg, M. & Ekoll, A. B., “Reduktionsfiske i Ringsjön 2005–2017. Fiskbeståndens utveckling och riktlinjer för fortsatta åtgärder”, Ringsjöns vattenråd, 2018. | ||
[68] | Meijer, M-L., de Boois, I., Scheffer, M., Portielje, R. & Hosper, H., “Biomanipulation in shallow lakes in The Netherlands: an evaluation of 18 case studies”, Hydrobiologica, 408/409, pp. 13–30, 1999. | ||
[69] | Hedrén, A. and the Municipality of Växjö, “Reduktionsfiske i Växjösjöarna – slutredovisning av ett LOVA-projekt 2016–2018”, Municipality of Växjö, Växjö, 2018. | ||
[70] | Bergqvist, B. et al, “Standardiserat elfiske i vattendrag – en manual med praktiska råd”, SLU Aqua, 2014. | ||
[71] | Søndergaard, M., Jeppesen, E., Lauridsen, T. L., Skov, C., van Nes, E. H., Roijackers, R., Lammens, E. & Portielje, R., “Lake restoration: successes, failures and long-term effects”, Journal of Applied Ecology, 44, pp. 1095–1105, 2007. | ||
[72] | Municipality of Jönköping, “Restaurering av Barnarpasjön”, 2014. [Online]. Available at: https://www.jonkoping.se/byggabomiljo/naturvardochskotselavgronomraden/vattenochvatmarker/overgodningivatten/barnarpasjonovergodning/restaureringavbarnarpasjon | ||
[73] | Gustafsson, A., Rydin, E. & Lindqvist, U., “Vattenkvalitet och plankton i Vallentunasjön 2017. Utvärdering av effekter av biomanipulering”, Naturvatten i Roslagen AB. Report 2018:4, 2018. | ||
[74] | Gächter, R. & Wehrli, B., “Ten Years of Artificial Mixing and Oxygenation: No Effect on the Internal Phosphorus Loading of Two Eutrophic Lakes”, Environ. Sci. Technol., pp. 3659–3665, 1998. | ||
[75] | Horppila, J., Holmroos, H., Niemistö, J., Massa, I., Nygrén, N., Schönach, P., Tapio, P. & Tammeorg, O., “Variations of internal phosphorus loading and water quality in a hypertrophic lake during 40 years of different management efforts”, Ecological Engineering, pp. 264–274, 2017. | ||
[76] | Cooke, G. D., Welch, E. B., Peterson, S. & Nichols, S. A., 2016. Restoration and management of lakes and reservoirs. CRC press. | ||
[77] | Beutel, M.W. & Horne, A., “A Review of the Effects of Hypolimnetic Oxygenation on Lake and Reservoir Water Quality”, Journal of Lake and Reservoir Management, pp. 285–297, 1999. | ||
[78] | Nürnberg, G. K., “Phosphorus release from anoxic sediments: what we know and how we can deal with it”, Limnética, 1994. | ||
[79] | Liboriussen, L., Søndergaard, M. & Jeppesen, E., “Sørestaurering i Danmark Del II: Eksempelsamling”, Danmarks Miljøundersøgelser, Aarhus University, vol. DMU no 636, p. 312 s, 2007. | ||
[80] | Schönach, P., Tapio, P., Holmroos, H., Horppila, J., Niemistö, J., Nygrén, N.A., Tammeorg, O. & Massa, I., “Persistency of artificial aeration at hypertrophic Lake Tuusulanjärvi: A sociohistorical analysis”, Ambio, pp. 865–877, 2017. | ||
[81] | Agstam-Norlin, O., Lannergård, E. E., Futter, M. N., & Huser, B. J., Optimization of aluminum treatment efficiency to control internal phosphorus loading in eutrophic lakes. Water Research, 185, 116150, 2020. | ||
[82] | Lürling, M., Waajen, G., & van Oosterhout, F., Humic substances interfere with phosphate removal by lanthanum modified clay in controlling eutrophication. Water research, 54, 78–88, 2014. | ||
[83] | Pilgrim, K. M., Huser, B. J., & Brezonik, P. L., A method for comparative evaluation of whole-lake and inflow alum treatment. Water research, 41(6), 1215–1224, 2007. | ||
[84] | Mehner, T., Benndorf, J., Kasprzak, P., & Koschel, R., Biomanipulation of lake ecosystems: successful applications and expanding complexity in the underlying science. Freshwater Biology, 47, 2453–2465, 2002. | ||
[85] | Hilt, S., Gross, E.M., Hupfer, M., Morscheid, H., Mählmann, J., Melzer, A., Poltz, J., Sandrock, S., Scharf, E., Schneider, S.A., & Weyer, K.V., Restoration of submerged vegetation in shallow eutrophic lakes: a guideline and state of the art in Germany. Limnologica, 36, 155–171, 2006. | ||
[86] | Jensen H.S., Andersen F.O., Importance of temperature, nitrate and pH for phosphate release from aerobic sediments of four shallow eutrophic lakes, Limnol. Oceanogr. 37: 577–589, 1992. |
Footnotes
[1] Water authorities, 2016. Åtgärder mot övergödning för att nå god ekologisk status – underlag till vattenmyndigheternas åtgärdsprogram. Report 2016:19.
[2] Huser B., Löfgren S. & Markensten H., 2016. Internbelastning av fosfor i svenska sjöar och kustområden – en kunskapsöversikt och förslag till åtgärder för Vattenförvaltningen. Swedish University of Agricultural Sciences Report 2016:6.
[3] Örebro County Administrative Board. “Från Skåne till Norrbotten: Identifiering av sjöar med förhöjd internbelastning.” Funded by a grant from the Swedish Agency for Marine and Water Management.
[4] A turnover time of 1 year means that it takes 1 year for the inflow of water to replace the entire water volume of the lake. A turnover time of 0.3 year means that it takes 4 months. In this example the inflow during the relatively dry conditions from May to August 2019 was merely 5% of the lake’s water volume corresponding to a turnover time of more than 5 years.
[5] Malmaeus J.M. & Håkanson L., 2004. Development of a Lake Eutrophication model. Ecological. Modelling 171, 35–63.
[6] Malmaeus J.M., Karlsson O.M., Lindgren D. & Eklund J., 2008. The optimal size of dynamic phosphorus models for Baltic coastal areas. Ecological modelling 216, 303–315.
[7] Malmaeus M., 2021. Att modellera internbelastning av fosfor i sjöar. Nya rutiner i LEEDS-modellen. IVL report C615.
[8] Phillips G., Lyche-Solheim A., Skjelbred B., Mischke U., Drakare S., Free G., Järvinen M., de Hoyos C., Morabito G., Poikane S. & Carvalho L. (2012). A phytoplankton trophic index to assess the status of lakes for the Water Framework Directive. Hydrobiologia 704 (1): 75–95.
[9] Malmaeus, M. & Karlsson, M. 2015 Fosfordynamik i Hjälmaren – resultat av simuleringar. IVL Swedish Environmental Research Institute. Report C72. https://www.ivl.se/publikationer/publikationer/fosfordynamik-i-hjalmaren.html
[10] Agstam-Norling, O., Lannergård, E.E., Rydin, E., Futter, M.N. & Huser, B.J., 2021. A 25-year retrospective analysis of factors influencing success of aluminum treatment for lake restoration, Water Research 200: 117267.
[11] Rydin, E. & Kumblad, L., 2019. Capturing past eutrophication in coastal sediments – towards water-quality goals, Estuarine, Coastal and Shelf Science 221: 184–188.
[12] Robb M., Greenop B., Goss Z., Douglas G. & Adeney J., 2003. Application of PhoslockTM, an innovative phosphorus binding clay, to two Western Australian waterways: preliminary findings, Hydrobiologia 494: 237–243.
[13] Zeller, M.A. & Alperin, M. J., 2021. The efficacy of PhoslockTM in reducing internal phosphate loading varies with bottom water oxygenation. Water Research 11: 100095.
[14] Renman, G., Renman, A. & Gustafsson, J. P., 2013. Reaktiva sorbent för fastläggning av fosfor i Östersjöns bottnar. BalticSea2020
[15] Zamparas, M. & Zacharias, I., 2014. Restoration of eutrophic freshwater by managing internal nutrient loads. A review. Science of the Total Environment, 496: 551–562.
[16] Blomqvist, S. & Björkman, E., 2014. Permanent inaktivering av fosfor i Östersjöns bottensediment. Forskningsrapport till BalticSea2020. Sammanställning av resultat framtagna juni 2012 till september 2013. Department of Ecology, Environment and Plant Sciences, Stockholm University.
[17] G. K. Nürnberg, 2007. Lake responses to long-term hypolimnetic withdrawal. Lake and Reservoir Management, 388–409.
[18] Nürnberg, G.K., 2020. Hypolimnetic withdrawal as a lake restoration technique: determination of feasibility and continued benefits. Hydrobiologia, vol. 847: 4487–4501.
[19] Swedish Agency for Marine and Water Management, 2018. Muddring och hantering av muddermassor – vägledning och kunskapsunderlag för tillämpningen (Report number 2018:19). Swedish Agency for Marine and Water Management, Gothenburg.
[20] Municipality of Jönköping, “Restaurering av Barnarpasjön”, 2014. [Online].
[21] Lindell, M., Carlsson, F., & Ekström, M., 2022. Återföring av näringsrika ytsediment till produktionsmark – erfarenheter av muddring, pelletsproduktion samt testodling. Jönköping County Administrative Board, Bulletin 2022:09.
[22] Herbertsson, J. Lågflödesmuddring i sjön Öljaren [Online]. Available: https://www.richwaters.se/vara-projekt/lagflodesmuddring-oljaren/. [Accessed on 23 January 2023.]
[23] Søndergaard, M., Jensen, J. P. & Jeppesen, E., 2003. Role of sediment and internal loading of phosphorous in shallow lakes”, Hydrobiologica, 506: 135–145.
[24] Bernes, C., Carpenter, S. R., Gårdmark, A., Larsson, P., Persson, L., Skov, C., Speed, J. D. M. & Van Donk, E., 2015. What is the influence of a reduction of planktivorous and bentivorous fish on water quality in temperate eutrophic lakes? Environmental Evidence 4:13.
[25] Franzén, F. & Svensson, M., 2022. Utvärdering av sociala och ekonomiska effekter av Rich Waters delprojekt. Published digitally at https://www.richwaters.se/utvardering-av-sociala-och-ekonomiska-effekter-av-rich-waters-delprojekt/
[26] Soutukorva, Å., Wallström, J., Ivarsson M. & Wallentin, E., 2017. Värdering av vattenförekomster i Stockholm. Anthesis Enveco AB, report 2017:5.
[27] Karlsson, M., Malmaeus, M., Baresel, C., Sivard, Å., Ericsson, T. & Grahn, O., 2012. Kostnadseffektivitet i åtgärder mot övergödning – Fallstudie Gävle fjärdar, IVL-rapport B2078.
[28] Karlsson, M., Malmaeus, M. & Rydin E., 2019. Åtgärder mot internbelastning av fosfor i Hjälmaren – kostnader, nytta och konsekvenser. IVL report C381.
[29] Section 15 of the Ordinance on state funding for local water management projects (2009:381). Government Offices of Sweden, Ministry of Climate and Enterprise.